Proteomic profiling of extracellular vesicles suggests Collectin10 as potential biomarker in relapsing head and neck squamous cell carcinoma
DOI: https://doi.org/10.47184/tev.2022.01.01Head and neck squamous cell carcinoma (HNSCC) is the 6th most common cancer worldwide. Developing new therapies has been ongoing for many decades, however, the 5-year overall survival rate remains comparably low and has not improved significantly. Treatment failure in HNSCC patients is common, especially in recurrences, and results in a poorer prognosis. Therefore, a better understanding of the disease is crucial to detect HNSCC recurrences at an early stage. HNSCC-associated extracellular vesicles (EVs), have been shown to suppress the immune system and thereby promote tumor progression. However, it is still unclear which pathways play a pivotal role in relapse or in remission. Contamination-free plasma EVs were purified using the EXÖBead® technology and EVs proteins underwent holistic proteome profiling by high-resolution mass spectrometry. COLEC10 appears to be the most regulated EVs protein and therefore has been chosen for further analysis and validation. Furthermore PanEV+ COLEC10+ and PanEV+ CD45Neg COLEC10+ of plasma EVs-EXÖBead complexes also showed a higher expression in relapsed patients compared to remission. Gene ontology analyses suggested that the relapse-upregulated proteins are significantly enriched in pathways for collagen trimer and chain formation, the complement system activation and in the tumor necrosis factor like domain superfamily related pathway. The remission-upregulated proteins were significantly enriched in early endosome or lysosomal membrane formation, membrane stability, mRNA stability and in ubiquitin protein ligase binding. According to common tissue RNA-seq database elevated COLEC10 gene has been associated with shorter survival in female patient and observed from different clinical cohort. Our results indicate that increased protein expression in EVs of patients with recurrent disease might contribute to disease progression with COLEC10 as potential biomarker in HNSCC.
Keywords: Extracellular vesicles, HNSCC, EVs proteomics, biomarkers and Collectin10
Introduction
Head and neck squamous cell carcinoma (HNSCC) is the 6th most common cancer worldwide [1]. Most HNSCC patients present at a locally advanced stage, and traditional treatments are challenging. Patients with advanced HNSCC frequently develop the relapsing disease [2]. Combination of therapies, such as surgery, radiation, chemotherapy, and target therapies, are usually used for advanced local diseases. However, up to 55 % of patients will still develop loco-regional recurrence or tumor progression with metastasis after combination therapies within two years [3]. As such, the five years overall survival rate remains very poor. Although salvage surgery, further radiation and immunotherapy are frequently used for recurrent HNSCC patients, most of cases are incurable [4–8]. Therefore, a better understanding of the pathogenesis is urgently needed to detect possible relapse.
The tumor microenvironment (TME) consists of secreted immunosuppressive heterogeneous molecules, such as IL-10, IL-8, TGF-β, tumor-related extracellular vesicles (TREXs) and so on [9–11]. TREXs are small extracellular vesicles (small EVs), 30–150 nm in diameter, made up of a lipid bilayer, and were found in all body fluids, such as blood, saliva, and urine of HNSCC patients [12–14]. Common EVs surface markers, such as CD9, CD63, and CD81 are also expressed on TREXs surface [15, 16]. To promote tumor progression, immune suppression, and angiogenesis, TREXs may include additional oncogenes, such as FasL and PD-L1, EGFR, TGFβ [17]. Circulating EVs derived from stromal fibroblasts can also induce TGFβ signaling even without TGFβ present [18]. It is reported that TGFβ type II receptor (TβRII) can be transferred from stromal fibroblasts to squamous cell carcinoma (SCC) by EVs [18]. TREXs also have regulative miRNAs as cargo [17], e. g. the exosomal miR-21 derived from hypoxia HNSCC cells induced normoxic cells to prometastatic phenotype [19] and miR-34a-5p derived from cancer-associated fibroblasts (CAFs) promote oral squamous cell carcinoma cell proliferation and metastasis [20]. The (detectable) number of exosomes from patients with active disease is significantly increased compared to healthy individuals [21]. Furthermore, HNSCC patients' plasma derived exosomes interfered CD4+ T cells activation by up-regulation of inhibitor genes and down-regulation of activation genes [22]. Exosomal PD-L1 expression is correlated to the HNSCC disease stage, and PD-L1high exosome suppresses CD8+ T cells activity and down-regulation of T cell activation marker CD69 [23]. Immuno-captured CD44v3 exosomes express the surface markers EpCAM, CD44v3, PD-L1, FasL, TGF-β1 and CSPG4 were significantly higher on CD44v3+ EVs-beads complex compared to CD44v3- EVs-beads complex [24]. Most of proteomics studies in HNSCC were focus on tumor progression and immune suppression. However, there is little literature available that investigates the role of EVs in the context of relapse and remission, even from tissue level [25]. Thus, there is an urgent and unmet need to analyze the complete protein contents in relapse and remission patients applying a holistic proteomics analysis. The following pilot study investigated the EVs protein contents by using galectin-based glycan recognition particles (EXÖBead) isolation and high-resolution mass spectrometry (HRMS). Proteomics approach can help us to understand which EVs’ proteins and pathway may be critical in relapsing HNSCC. Future studies with alarger cohorts and EVs functional assays are needed to better understand the role of these first identified target proteins.
Material & Methods
Patient sample collection
HNSCC patients from the department of otorhinolaryngology of the Basel University Hospital, Switzerland, with relapse or remission. Informed consent was obtained from all patients. Ethical approval was issued by the ethical commission of the northwest and central Switzerland (project-ID 2020-02173). A standardized peripheral venous blood collection was performed (EDTA, S-Monovette, Sarstedt, Germany). Whole blood was further centrifuged at 800 g for 10 minutes at room temperature to remove cells and cell debris. Platelet-rich plasma (PRP) was collected into new tubes. Platelet-poor plasma (PPP) was generated from PRP by additional 10,000 g centrifugation at 4 °C for 30 min, aliquoted, and stored at -80 °C [21, 26, 27].
Galectin-based glycan recognition particles EVs isolation, EXÖBead
The EXÖBead are magnetic beads coated with galectins for the isolation of EVs. The basis of this was the detection of N-linked glycoproteins on EVs, in particular galectin-3-binding protein (LGALS3BP) (European patent number: EP3602055; US patent number: US 11079374 B2), which was found on EVs from ovarian cancer cells [28, 29]. EXÖBead has previously been used for pure EV isolation in experimental atherosclerosis [30] and amyotrophic lateral sclerosis [31]. Our recent HNSCC results also showed that HNSCC patient’s plasma EVs can be isolated by EXÖBead with high EVs markers, low lipoprotein contamination [32]. Eluted
HNSCC patient’s plasma EVs represented high immune suppression activity [32].
Proteins preparation for proteomics
2 ml platelet-poor plasma (PPP) were diluted directly in 2 ml of PBS and incubated with 100 μl of EXÖBead (1 μm, 1x109 particles/ml, Biovesicle Inc., Taipei, Taiwan) for 1 hour at 37 °C. EV-EXÖBead complexes were washed twice with 1 ml 0.1M glycine (Merck KGaA, Darmstadt, Germany) in PBS. EV-EXÖBead complexes were resuspended in 50 μl of 1x Laemmli Sample Buffer in 1x RIPA buffer (4x Laemmli Sample Buffer, Bio-Rad Laboratories, Hercules, CA, USA) (10X RIPA Buffer, Abcam, Cambridge, MA, USA) with 2-mercaptoethanol (Merck KGaA, Darmstadt, Germany). Laemmli Sample Buffer with EV-EXÖBead complexes were put into -80 °C for overnight incubation. Laemmli Sample Buffer with EV-EXÖBead complexes was boiled at 70 °C for 10 minutes. Boiled Laemmli Sample Buffer with EV-EXÖBead complexes was sonicated in 4 °C for 5 minutes. Sonicated samples were centrifugation at 10,000 g at 4 °C for 30 minutes. Sonicated samples were stood on the magnetic separation rank to remove beads before sending proteins within the sample buffer to the proteomics core facility.
High-resolution mass spectrometry (HRMS)
According to standard procedures, in-gel trypsin digestion of all plasma EV samples was performed [33]. Briefly, the samples were separated on a Nu-PAGE™ 4 %–12 % Bis‐Tris protein gel (Thermo Fisher Scientific) for about 1 cm. Subsequently, the accumulated and not size‐separated single protein band per sample was cut out, reduced (50mM dithiothreitol), alkylated (55mM chloroacetamide), and digested overnight with trypsin (Trypsin Gold, mass spectrometry grade, Promega). The dry peptide samples were resuspended in 25 μl buffer A (2 % acetonitrile, 0.1 % formic acid in HPLC grade water), of which 5 μl were injected per MS measurement.
LC-MS/MS measurements were carried out on a Dionex Ultimate 3000 RSLCnano system coupled to a Q-Exactive HF-X mass spectrometer (Thermofisher Scientific, Bremen). Injected peptides were delivered to a trap column (ReproSil-our C18-AQ, five μm, Dr. Maisch, 20 mm × 75 μm, self-packed) at a flow rate of 5 μL/min in 100 % solvent A (0.1 % formic acid in HPLC grade water). After 10 minutes of loading, peptides were transferred to an analytical column (ReproSil Gold C18-AQ, three μm, Dr. Maisch, 450 mm × 75 μm, self-packed) and separated using a 50 min gradient from 4 % to 32 % of solvent B (0.1 % FA, 5 % DMSO in acetonitrile) in solvent A (0.1 % FA, 5 % DMSO in HPLC grade water) at 300 nL/min flow rate. The Q-Exactive HF-X mass spectrometer was operated in data-dependent acquisition (DDA) and positive ionization mode. MS1 spectra (360–1300 m/z) were recorded at a resolution of 60k using an automatic gain control (AGC) target value of 3e6 and a maximum injection time (maxIT) of 45 msec. Up to 18 peptide precursors were selected for fragmentation. Only precursors with charge states 2 to 6 were selected, and dynamic exclusion of 25 sec was enabled. Peptide fragmentation was performed using higher-energy collision-induced dissociation (HCD) and normalized collision energy (NCE) of 26 %. The precursor isolation window width was set to 1.3 m/z. MS2 Resolution was 15.000 with automatic gain control (AGC) target value of 1e5 and a maximum injection time (maxIT) of 25 msec (complete proteome).
Protein label-free quantification (LFQ)
Peptide identification and quantification were performed using the software MaxQuant (version 1.6.3.4) [34] with its built‐in search engine Andromeda [35].MS2 spectra were searched against the human protein database from Uniprot (UP000005640, downloaded 04.05.2020) supplemented with common contaminants (built‐in option in MaxQuant). Trypsin/P was specified as a proteolytic enzyme, and Carbamidomethylated cysteine was set as a fixed modification. Oxidation of methionine and acetylation at the protein N‐terminus were defined as variable modifications. Results were adjusted to a 1 % false discovery rate on peptide spectrum match level and protein level employing a target‐decoy approach using reversed protein sequences. LFQ intensity were log10 transformed [36]. Proteins constantly detected in at least four technical replicates in one of the remission and relapse groups were retained. Student t-test was used to identify proteins differentially expressed between the two groups. Fisher's exact test was used in the enrichment analysis. The protein domain database interpro [37–39] and GO were used in the enrichment analysis [40]. Statistical data analyses were performed using R (version 3.6.3) [41]. Candidate proteins networks were visualized via STRING v11 [42].
COLEC10 staining and with HNSCC biomarkers staining
0.75 ml of platelet-poor plasma (PPP) were directly in 1 ml PBS and incubated with 25 μl of EXÖBead for 1 hour at 37 °C. EV-EXÖBead complexes were washed twice with 1 ml 0.5 % EVs-free BSA (bovine serum albumine: SERVA Electrophoresis GmbH, Heidelberg, Germany) in PBS (EVs-free preparation: 100,000 g, 4 °C for overnight centrifugation) while magnetic EXÖBeads were kept in the tube by using a magnet (Biovesicle Inc., Taipei, Taiwan). 1 ml of 10 % EV-free fetal calf serum in PBS (EVs-free preparation: 100,000 g, 4 °C for overnight centrifugation) is also incubated with EXÖBead as a negative control (EF-EXÖBead complex).
For COLEC10 surface and intracellular staining, 0.5 μl of Alexa Fluor® 488 anti-COLEC10: BS-8033R (1 μg/μl, Bioss, Woburn, USA) were added in 100 μl 0.5 % EVs-free BSA in PBS. For COLEC10 with HNSCC biomarkers, PanEV antibodies were used as a positive EV marker instead of three individual antibodies. PE-PanEV antibody was pre-mixed with 1 μl of PE anti-CD63: H5C6 clone, 1 μl of PE-CD81: 5A6 clone, and 1 μl of PE-CD9: HI9a clone (Biolegend, San Diego, USA). The 100 μl of antibodies master mix were contained the PanEV marker, two disease-specific biomarkers, common leukocyte antigen CD45, and COLEC10. The mixture was set up of 3 μl of PE-PanEV antibodies, 0.5 μl of Alexa Fluor® 488 anti-COLEC10: BS-8033R (1 μg/μl, Bioss, Woburn, USA), 1.5 μl of Brilliant Violet 421™ anti-human CD45 Antibody: HI30 (Biolegend, San Diego, USA), 1.5 μl of APC anti-PD-L1 antibody: MIH1 (ThermoFisher Scientific, Massachusetts, USA) and 1.5 μl of APC-Cy7 Mouse Anti-Human EpCAM/CD326 antibody: 9C4 (APC-Cy7 Mouse Anti-Human EpCAM/CD326 antibody).
100 μl of antibodies master mix was each incubated with plasma EV-EXÖBead complexes as well as with EF-EXÖBead complexes for surface staining. Incubation was lasted for 1 hour at 25 °C. After antibody incubation, the EV-EXÖBead and EF-EXÖBead complexes were washed twice with 1 ml of 0.5 % EV-free BSA in PBS, while the magnetic EXÖBead were held in the tube using a magnet. After surface staining, plasma EV-EXÖBead complexes and EF-EXÖBead complexes were then fixed by 200 μl of 2 % paraformaldehyde in PBS for 10 minutes at 25 °C, penetrated by 200 μl of 0.5 % Tween-20 in PBS for 10 minutes at 25 °C and blocked by 200 μl of 10 % EV-free fetal calf serum in PBS (EVs-free preparation: 100,000 g, 4 °C for overnight centrifugation) for 30 minutes at 25 °C. For further intracellular staining, fixed/penetrated/surface antibodies-stained plasma EV-EXÖBead complexes and EF-EXÖBead complexes were further stained with 200 ul of 0.5 % EV-free BSA in PBS with 0.5 μl of Alexa Fluor 488 anti-COLEC10: BS-8033R (1 μg/μl, Bioss, Woburn, USA) at 37 °C for 1-hour incubation. Plasma EV-EXÖBead and EF-EXÖBead complexes surface/intracellular stained with antibodies were subsequently prepared on the BD LSR Fortessa™ (BD Biosciences), and the data were analyzed using FlowJo software (Tree Star, Ashland, Oregon, USA).
Gating strategy
Antibodies-stained EF-EXÖBead complexes was served as a non-EV control and gating was based on FlowJo software (Tree Star, Ashland, Oregon, USA). "PanEV+ and COLEC10+orNeg" on Plasma EV-EXÖBead complexes was gated which based on the background signal from EF-EXÖBead complexes. The PanEVNeg COLEC10+, PanEV+ COLEC10+, PanEV+ COLEC10Neg, and PanEVNeg COLEC10Neg on plasma EV-EXÖBead complexes based on plasma EV-EXÖBead complexes were expressed as percentages of beads.
For the distinguishing of tumor EVs/immune cells EVs, PanEV+ and CD45Neg on plasma EV-EXÖBead complexes was gated which based on the background signal from EF-EXÖBead complexes. The PanEV+ CD45Neg COLEC10+, PanEV+ CD45+ COLEC10+, PanEVNeg CD45+ COLEC10+ and PanEVNeg CD45Neg COLEC10+ on plasma EV-EXÖBead complexes were expressed as percentages of beads.
For two positive populations, PanEV+ CD45Neg plasma EV-EXÖBead complexes were also gated by EpCAM+/Neg or PD-L1+/Neg and then further gated COLEC10+/Neg also based on the background signal from EF-EXÖBead complexes. PanEV+ CD45Neg EpCAMNeg COLEC10+, PanEV+ CD45Neg EpCAM+ COLEC10+, PanEV+ CD45Neg EpCAM+ COLEC10Neg, PanEV+ CD45Neg EpCAMNeg COLEC10Neg, PanEV+ CD45Neg PD-L1Neg COLEC10+, PanEV+ CD45Neg PD-L1+ COLEC10+, PanEV+ CD45Neg PD-L1+ COLEC10Neg and PanEV+ CD45Neg PD-L1Neg COLEC10Neg on plasma EV-EXÖBead complexes were expressed as percentages of beads.
For the triple-positive CD45Neg EV subpopulation gating strategy, PanEV+ CD45Neg EV-nbvCOLEC10+ is also based on background signal from EF-EXÖBead complexes. The final four triple-positive populations of PanEV+ CD45Neg EpCAM+ PD-L1Neg COLEC10+, PanEV+ CD45Neg EpCAM+ PD-L1+ COLEC10+, PanEV+ CD45Neg EpCAMNeg PD-L1+ COLEC10+ and PanEV+ CD45Neg EpCAMNeg PD-L1Neg COLEC10+ on plasma EV-EXÖBead complexes were expressed as percentages of beads. Two-way ANOVA calculated significance with a Two-stage linear step-up procedure of Benjamini, Krieger, and Yekutieli comparison by Prism version 8 (GraphPad, California, USA).
Results
LC-MS/MS-based proteomics
We profiled the EV protein composition derived from relapse or remission HNSCC patients using LC-MS/MS-based proteomics. The EV isolation process via EXÖBead was performed in MISEV compliancy [43]. The isolated HNSCC patients’ plasma EVs showed high purity, high concentrations, high integrity, essential positive EV surface markers, low lipoprotein contamination, and still keep their immune suppression activity, as shown previously [32]. We identified 436-491 EV proteins in the four relapse patient samples (four technical replicates, p-value < 0.1 and fold change > 1.5; Fig 1A), and 436-537 proteins in the five remission patient samples, applying the same filter criteria (Fig. 1A).
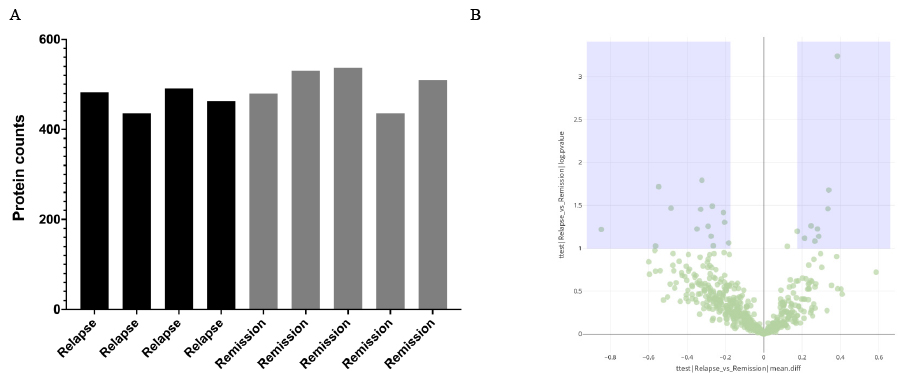
Figure 1: Proteins detected in extracellular vesicles derived by HNSCC relapsed or remission patients.
Bar plot showed the total protein counts in each sample with the filter criteria at least 4 technical replicates (A). Scatter plot showed that the log-transformed fold-change of each protein is plotted against the log-transformed fold-change. The purple rectangles were marked high significantly different proteins. Significance was calculated by Student t-test. Significance different criteria was set at p-value < 0.1 and fold change > 1.5 (B).
Common EVs markers, according to the MISEV guidelines, such as CD9, CD63, CD81, TSG101, Rab family and Alix can also be found from our proteomics result.
To further understand the proteome difference between relapse and remission samples, we used the student t-test to identify proteins significantly differentially expressed between the two groups. We identified COLEC10, COLEC11, C1QA, C1QB, KLK14, C1QC, FA10, KLK14, PA2G4 and CO3 proteins overexpressed in the relapse samples and CAH1, CAH2, 4F2, HS71B/HS71A, HSP7C, LAMP1, ENPL/ENPLL, 1433B, RAB5C, B2MG, RS27A/RL40/UBB/UBC, TP4A2, UBS3B and RAB21 proteins overexpressed in the remission group (p-value < 0.1 and fold change > 1.5; Fig. 1B). Over-representation GO analyses suggested that the relapse-upregulated proteins are significantly enriched in pathways for collagen trimer and chain formation, the complement system activation and in TNF like domain superfamily related pathway, and these may be important in relapse progression (Fig. 1; Table 1). The GO analyses in the remission-upregulated proteins are significantly enriched in early endosome or lysosomal membrane formation, membrane stability, mRNA stability and in ubiquitin protein ligase binding. These pathways suggested that endosome, lysosome activity and protein or mRNA degradation pathway may be important in the HNSCC remission group (Fig. 1; Table 2).
Table 1 and table 2: Top 10 hits and most significant pathways resulting from protein enrichment and gene ontology (GO) analysis.
Table 1 (relapse protein) | overlap_ids | p.value | p.adjusted |
---|---|---|---|
GO_GO:0005581_C:collagen trimer | C1QA, C1QB, C1QC, COLEC10, COLEC11 | 0.0000 | 0.0000 |
InterPro_IPR008160_Collagen. | C1QA, C1QB, C1QC, COLEC10, COLEC11 | 0.0000 | 0.0000 |
GO_GO:0006956_P:complement activation | CO3, C1QA, C1QB, C1QC, COLEC10, COLEC11 | 0.0000 | 0.0000 |
InterPro_IPR001073_C1q_dom. | C1QA, C1QB, C1QC | 0.0000 | 0.0003 |
InterPro_IPR008983_Tumour_necrosis_fac-like_dom. | C1QA, C1QB, C1QC | 0.0001 | 0.0004 |
GO_GO:0098794_C:postsynapse | C1QA, C1QB, C1QC | 0.0005 | 0.0026 |
GO_GO:0005576_C:extracellular region | CO3, C1QA, C1QB, C1QC, COLEC10, COLEC11, FA10, KLK14, PA2G4 | 0.0008 | 0.0034 |
GO_GO:0006958_P:complement activation, classical pathway | CO3, C1QA, C1QB, C1QC | 0.0013 | 0.0052 |
GO_GO:0030449_P:regulation of complement activation | CO3, C1QA, C1QB, C1QC | 0.0016 | 0.0058 |
InterPro_IPR016186_C-type_lectin-like/link_sf. | COLEC10, COLEC11 | 0.0024 | 0.0070 |
Table 2 (remission protein) | overlap_ids | p.value | p.adjusted |
---|---|---|---|
GO_GO:0005765_C:lysosomal membrane | 4F2;HSP7C/HSP72;LAMP1;RAB5C;RS27A/RL40/UBB/UBC | 0.0001 | 0.0046 |
GO_GO:0042470_C:melanosome | 4F2;HSP7C/HSP72;LAMP1;ENPL/ENPLL;1433B;RAB5C | 0.0001 | 0.0046 |
GO_GO:0043488_P:regulation of mRNA stability | HS71B/HS71A;HSP7C/HSP72;1433B;RS27A/RL40/UBB/UBC | 0.0002 | 0.0046 |
GO_GO:0005829_C:cytosol | CAH1;CAH2;4F2;HS71B/HS71A;HSP7C/HSP72; | 0.0003 | 0.0065 |
GO_GO:0042026_P:protein refolding | HS71B/HS71A;HSP7C/HSP72;B2MG | 0.0005 | 0.0072 |
GO_GO:0031901_C:early endosome membrane | RAB5C;B2MG;RAB21 | 0.0020 | 0.0265 |
GO_GO:0031625_F:ubiquitin protein ligase binding | HS71B/HS71A;HSP7C/HSP72;RS27A/RL40/UBB/UBC;UBS3B | 0.0026 | 0.0293 |
GO_GO:0019899_F:enzyme binding | HS71B/HS71A;HSP7C/HSP72;LAMP1;1433B | 0.0030 | 0.0298 |
GO_GO:0005737_C:cytoplasm | CAH1;CAH2;HS71B/HS71A;HSP7C/HSP72; | 0.0055 | 0.0398 |
GO_GO:0004725_F:protein tyrosine phosphatase activity | TP4A2;UBS3B | 0.0059 | 0.0398 |
Plasma EVs’ COLEC10 validation and clinical database research
COLEC10 was the most significant differentially expressed EVs’ protein in the relapse compared to the remission group (p-value 0.0006; Fig. 2A).
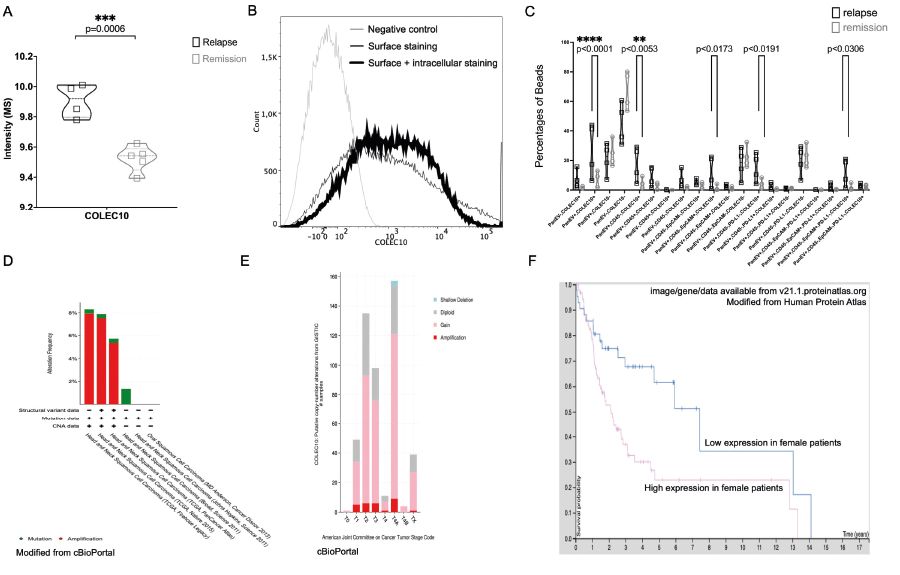
Figure 2: Collectin10 in extracellular vesicles as a potential biomarker in relapsing head neck cancer.
Violin plot showed the comparison of Label-Free Quantification (LFQ) intensity between COLEC10 expression in relapsed or remission patients’ plasma EVs. Significance was calculated by Student t-test (A).
Histogram plot showed COLEC10 expression on or inside patients’ plasma EVs-EXOBead complex. Negative controls showed in gray line, surface staining only showed in dashed line and surface plus intracellular staining showed in solid line (B).
Violin plot showed PanEV+/Neg and COLEC10+/Neg, PanEV+/Neg, CD45+/Neg and COLEC10+, PanEV+/Neg, CD45Neg, EpCAM+/Neg and COLEC10+/Neg, PanEV+/Neg, CD45Neg, PD-L1+/Neg and COLEC10+/Neg and PanEV+/Neg, CD45Neg, PD-L1+/Neg, EpCAM+/Neg and COLEC10+ of plasma EVs-EXOBead® complex (C). Significance was calculated by Two-way ANOVA with Two-stage linear step-up procedure of Benjamini, Krieger and Yekutieli method.
Bar plot of cBioPortal database showed copy number profile from different clinical datasets (D) and different disease stages (E). A survival curve gained from Human Protein Atlas database showed that a high expression of COLEC10 reduces cumulative survival of HNSCC female patients (p = 0.0040) (F).
To validate whether COLEC10 is a highly concentrated protein plasma EVs of relapse patients, we used beads-base flow cytometry (BFC) with surface/intracellular staining. BFC showed that COLEC10 is expressed on the EVs surface (Median fluorescence intensity, MFI: 750.2) and surface plus intracellular (MFI: 1052.2) (Fig. 2B). To understand whether relapse patients secreted more COLEC10+ EVs in plasma, we further intracellularly stained PanEV, CD45, EpCAM, PD-L1, and COLEC10 with relapse (n=4) and remission patients (n=4) by BFC. PanEV+ COLEC10+ of plasma EV-EXÖBead complex was significantly higher in relapsed patients compared to remission patients (p<0.0001) (Fig. 2C). Interestingly, we also found that PanEV+ CD45Neg COLEC10+ of plasma EV-EXÖBead complexes was significantly higher in relapsed patients (p<0.0053) compared to remission (Fig. 2C). PanEV+ CD45Neg EpCAM+ COLEC10+, PanEV+ CD45Neg PD-L1Neg COLEC10+, PanEV+ CD45Neg EpCAMNeg PD-L1Neg COLEC10+ of plasma EV-EXOBead® complex were also higher in relapse group (p<0.05, not discovered significant different) (Fig. 2C). However, we did not observe any difference from PanEV+ CD45Neg EpCAM+ COLEC10Neg, PanEV+ CD45Neg PD-L1+ COLEC10Neg of plasma EV-EXOBead® complexes between relapse or remission. These data suggest that COLEC10 in EV may serve as a potential biomarker instead of traditional HNSCC biomarkers, EpCAM and PD-L1.
To understand if COLEC10 abundance correlates with disease progression, we further screened clinical databases from cBioPortal for Cancer Genomics (https://www.cbioportal.org/) [44, 45] and the Human Protein Atlas v21.1 (https://www.proteinatlas.org/) [46–48]. Three out of six HNSCC clinical studies showed that gene amplification of COLEC10 were from cBioPortal databases (8.30 %, 7.89 %, and 5.74 %) (Fig. 2D) [44, 45]. From Genomic Identification of Significant Targets in Cancer (GISTIC) results, 59.18 % of T1, 64.44 % of T2, 71.43 % of T3, 54.55 % of T4, and 71.34 % of T4a patients represented a gain of COLEC10 gene (Fig. 2E) [44, 45]. In the Human Protein Atlas database (image/gene/data available from v21.1.proteinatlas.org), 5-year survival data showed that 23 % of COLEC10 high expression HNSCC female patients were significantly lower than 62 % of COLEC10 common expression HNSCC female patients (p=0.0040) (Fig. 2F) [46–48].
Discussion
This study identified some protein candidates which could serve as potential biomarkers for HNSCC. Eight proteins were significantly higher concentrated in EV samples from relapse patients, which are associated with Collagen, complement, and TNF related pathways. In EV samples from a remission group of patients, 14 proteins were significantly higher abundant, which are connected to the endosome/lysosome pathways. By comparison, COLEC10 in plasma EVs was among the most regulated proteins between both groups and showed a significantly higher concentration in relapse patients.
Collectin (COLEC, also called collagenous C-type lectins) is a C-type lectin family that contains a collagen-like triple-helical domain and Ca2+-dependent carbohydrate recognition domain (CRD). The Human Collectin family includes surfactant-associated protein A (SP-A), surfactant-associated protein D (SP-D), MBL, CL-L1 (COLEC10), CL-K1 (COLEC11), and CL-P1 (COLEC12). Ishita Choudhary et al. showed that exosomal surfactant-associated protein A1 (SP-A1/SFTPA1/COLEC4) and surfactant-associated protein D (SP-D/SFTPD/COLEC7) were higher in air-exposed mice compared to ozone‐exposed mice an inflammatory condition [49]. B. Sandfeld-Paulsen et al. observed that membrane bound proteins, SP-A and SP-D were also detected from non‐small cell lung cancer patients’ plasma derived exosomes by EV microarray [50]. Besides exosomes/EVs’ proteomics evidences, COLEC family have been also investigated by histology or cellular analysis. Recently various publications showed protein biomarkers in EVs from cancer patients. Tsutsumida et al. showed that lung adenocarcinoma patients with poor outcomes had lower SP-A and higher MUC1 expression [51]. Atsushi Mitsuhashi et al. showed that overexpression of SP-A reduced tumor progression by M1 macrophages recruitment in an experimental/xenograft animal study [52]. Juhi Kumar et al. observed that high expression of the SP-D gene was correlated to unfavorable prognosis, worse disease outcomes with poor overall and progression-free survival. SP-D protein was also expressed in circulating ovarian tumor cells in three patients [53]. However, Junru Li et al. showed that a lack of Mannose-binding lectin (MBL) promotes tumor progression and increases the myeloid-derived stem cells (MDSCs) population in hepatocellular carcinoma animal study [54]. Chenkai Huang et al. observed that COLEC10, one of the hub genes from the TCGA and GEO database, was associated with overall survival (p=0.008) [55]. Interestingly, our proteomics data (Fig. 1C&D) and validation results (Fig. 2 A-C) also revealed an over-expression of COLEC10 can be observed in relapse patients' plasma EVs. RNAseq database (from the human Protein Atlas) also showed that overexpression of COLEC10 in female patients has a lower 5-year survival rate. These results suggested that COLEC10 may play an important role in relapse patients. However, the function of COLEC10 in plasma EVs is still not fully understood. Future studies will be needed to perform functional assays and detect plasma EVs' COLEC10 in more patients.
In conclusion, proteomic analysis of plasma EVs from relapse and remission HNSCC patients identified differential enrichment in vesicular protein contents. COLEC10 appeared to be the most promising biomarker candidate protein in the relapse group, and could link disease progression and survival rate to advanced disease status. This could lead to a more aggressive diagnostic workup in patients with high COLEC10 plasma levels to detect the relapse to a timepoint as early as possible. Furthermore, additional studies with bigger patient cohorts are needed to confirm the first findings and validate these protein biomarkers.
Data availability
The mass spectrometry proteomics data have been deposited to the ProteomeXchange Consortium via the PRIDE partner repository with the dataset identifier PXD033898.
Acknowledgements
We thank Franziska Hackbarth and Verena Breitner for technical assistance at BayBioMS, as well as Miriam Abele for mass spectrometric support. We also thank Dr. Benedikt Kirchner for pathway analysis at TUM School of Life Sciences, Technical University of Munich, Freising, Germany.