Characterization of two novel neutral sphingomyelinase 2 inhibitors in endosomal sorting and extracellular vesicle biogenesis
DOI: https://doi.org/10.47184/tev.2022.01.02Sphingomyelinase hydrolyzes the phosphodiester bond of the sphingomyelin to ceramide and phosphorylcholine and have been involved in extracellular vesicle (EV) biogenesis and more recently in membrane repair. Here we describe an initial testing of two recently discovered neutral sphingomyelinase 2 (nSMase2) inhibitors ((R)-(1-(3-(3,4-dimethoxyphenyl)-2,6-dimethylimidazo[1,2-b]pyridazin-8-yl)pyrrolidin-3-yl)-carbamate (PDDC) and 2,6-dimethoxy-4-[4-phenyl-5-(2-thienyl)-1H-imidazol-2-yl]phenol (DPTIP)). PDDC and DPTIP show differential effects on cell viability, and EV marker secretion, indicating that side effects of these inhibitors on lysosomal and autophagic degradation pathways need to be considered. Moreover, similar to commonly used nSMase2 inhibitor GW4869, cell type specificity seems to play a role in the endosomal trafficking routes that can be explored to unravel mechanisms of specific EV biogenesis and secretion pathways.
Keywords: Exosomes, sphingomyelindiesterase 3 (SMPD3) inhibitor, autophagy
Introduction
Sphingomyelinase or sphingomyelindiesterase (SMPD), a member of the DNase-I superfamily of enzymes, hydrolyzes the phosphodiester bond of the sphingomyelin to generate two products: ceramide and phosphorylcholine (Airola & Hannun, 2013). Ceramide acts as a lipid signaling molecule in multiple cellular signaling pathways including cell growth, differentiation, and apoptosis (Arana et al, 2010). Six sphingomyelinases have been identified in mammalian cells and are categorized based on their pH optima of activity into acid, neutral, and alkaline sphingomyelinases. In mammals, four neutral sphingomyelinases (nSMases) have been identified, nSMase1 (encoded by SMPD2) (Tomiuk et al, 1998; Sawai et al, 1999), nSMase2 (encoded by SMPD3) (Hofmann et al, 2000), nSMase3 (SMPD4) (Krut et al, 2006), and mitochondrial associated nSMase (MA-nSMase; SMPD5) (Wu et al, 2010).
NSMase2 is a 655 amino acids long protein with a molecular weight of 71 kDa. It is a membrane-associating enzyme with an N-terminal domain and a C-terminal catalytic domain (Marchesini et al, 2003). NSMase2 is described as the major sphingomyelin hydrolyzing enzyme with its overexpression resulting in a significant decrease in sphingomyelin levels with a concomitant increase in ceramide levels in MCF-7 cells (Marchesini et al, 2003) and primary hepatocytes (Karakashian et al, 2004). At the cellular level, although nSMase2 was described to be localized mainly at the plasma membrane (Airola & Hannun, 2013), later studies have shown that it localizes to different subcellular compartments including the Golgi (Stoffel et al, 2016; Back et al, 2018), the endoplasmic reticulum, nucleus, and endosomes (Shamseddine et al, 2015; Airola & Hannun, 2013). NSMase2 is described as the major stress-induced ceramide-producing enzyme. For example, nSMase2 was implicated in the anti-cancer drug daunorubicin-induced cell death by generating ceramide (Ito et al, 2009). Additionally, nSMase2 was shown to play a role in stress-induced bronchial and lung injury in pulmonary diseases by inducing apoptosis (Levy et al, 2006). In line with this study, nSMase2 is also involved in inducing apoptosis by generating ceramide in bronchial epithelial cells exposed to cigarette smoke (Levy et al, 2009).
NSMase2 is involved in intraluminal vesicles (ILVs) budding into late endosomes or multivesicular bodies (MVBs) (Trajkovic et al, 2008). Fusion of these with the plasma membrane results in exosomes or small extracellular vesicles (sEV) secretion (Van Niel et al, 2018). Ceramide generates negative membrane curvature required for ILV budding into the MVB lumen (Trajkovic et al, 2008). A previous study has shown that ceramide produced by nSMase2 activity is further metabolized into sphingosine-1 phosphate (S1P) which activates its cognate S1P receptor (S1PR) on the MVB membrane that drives CD63-cargo loading into ILVs for sEV secretion (Kajimoto et al, 2013). In agreement with these studies, nSMase2 activity is required for packing prion proteins into ILVs for their eventual release on sEV and thereby implicates a potential role for nSMase2 in neurodegenerative disorder progression (Guo et al, 2017).
Therefore nSMase2 activity inhibition has become a major experimental approach for studying the functional roles of EVs. So far, inhibition is mediated by either siRNA knock-down or pharmacological inhibition with nSMase2-specific inhibitor GW4869. In 2002, GW4869 was identified as a specific nSMase2 inhibitor in a high-throughput screen. First anti-apototic effects were the major focus of early studies (Wiegmann et al, 1994), in 2008, it was revealed that nSMase2 inhibition was strongly reducing EV secretion (Trajkovic et al, 2008). Today GW4869 is a commonly used inhibitor of nSMase2 acitivity that leads to reduction of EV secretion in several cell lines and models (Catalano & O’Driscoll, 2020). The noncompetitive inhibitor has a high IC50 of 1 μM and a low solubility (DMSO : 0.1 mg/ml (0.17 mM)). However nSMase2 inhibition also affects EV populations in a differential manner, in some cell lines it can affect EV size and lipid composition, increasing the release of larger microvesicles, while inhibiting the secretion of exosomes (Menck et al 2017). Moreover, high doses of vehicle, i. e. DMSO addition results in several side effects when analyzing EV secretion such as an increase in membrane fluidity and also artefacts in microscopy (Catalano & O’Driscoll, 2020). Therefore EV experiments are often complemented by SMPD3 KD by siRNA, which phenocyopies several but not all aspects of GW4869 treatment. As inhibitors are faster in their effects and applicable in vivo, , novel inhibitors of nSMase2 activity could help to shed light on the detailed function of nSMase2 in cellular processes. Recently, the group of B. Slusher developed two new nSMase2 inhibitors, (R)-(1-(3- (3,4-dimethoxyphenyl)-2,6-dimethylimidazo [1,2-b]pyridazin-8-yl)pyrrolidin-3-yl)-carbamate (PDDC) and 2,6-dimethoxy-4-[4-phenyl-5- (2-thienyl)-1H-imidazol-2-yl]phenol (DPTIP), which were shown to dose‐dependently inhibit astrocyte‐derived extracellular vesicles both in vitro (0.03–30 μM) and in vivo (30 mg/kg) (Rojas et al, 2018; Šála et al, 2020) .
Here, we tested the effects of nSMase2 inhibitors PDDC and DPTIP on EV secretion from HCT116 colon cancer cells and HeLa cervical cancer cells. The results from our initial study show that PDDC and DPTIP differentially affect cell viability and EV marker secretion and moreover indicate that these inhibitors have previously undescribed effects on lysosomal and autophagic degradation pathways, that need to be considered. Similar to GW4869, cell type specificity seems to play a role that can be explored to unravel mechanisms of EV biogenesis and secretion.
Results
DPTIP and PDDC are novel nSMase2 inhibitors
Due to the importance of understanding membrane turnover and trafficking in EV biogenesis, we tested the effects of nSMase2 inhibitors PDDC and DPTIP at a concentration of 10 μM on EV secretion from HCT116 colon cancer cells and HeLa cervical cancer cells in agreement with previous pharmacological active concentrations between 1–30 μM in astrocytes cell cultures (Rojas et al, 2018; Šála et al, 2020). Using a differential centrifugation protocol, the final pellet after 100,000g centrifugation (P100) was analyzed for the presence of EV markers by western blot in HCT116 in three independent experiments. Both inhibitors showed a strong and significant reduction of EV markers secretion Alix, CD63 and Synthenin in P100, with only mild accumulation of EV markers Alix in the cell extract (Fig.1 A-D).
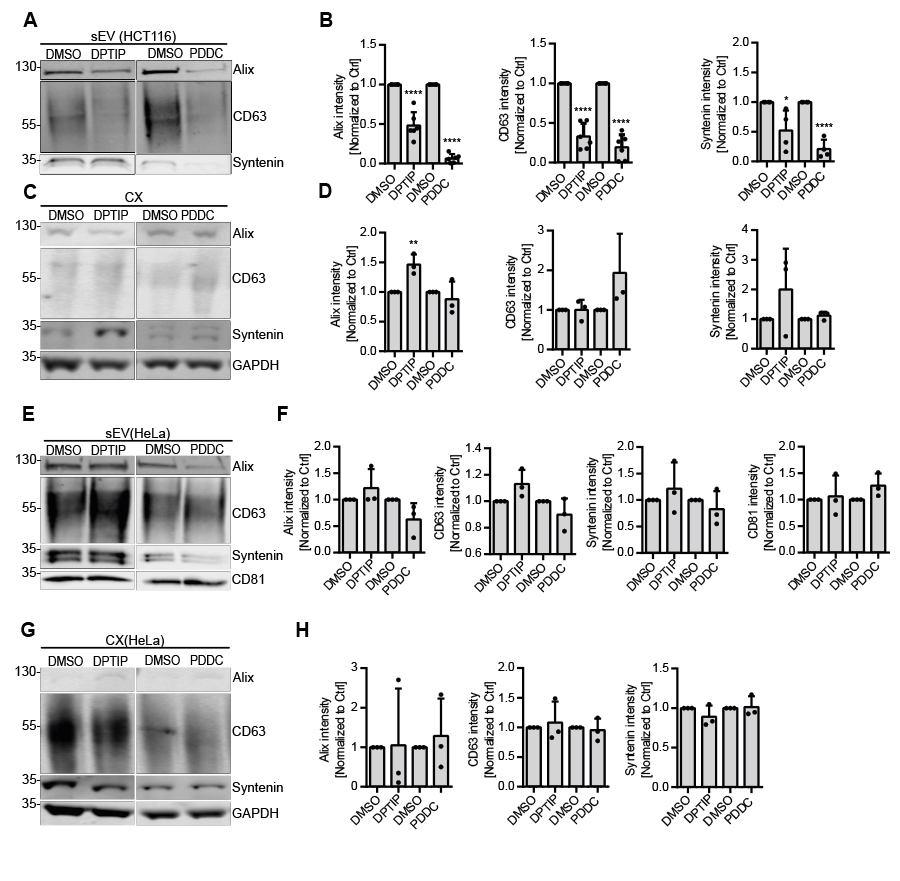
Figure 1: DPTIP and PDDC are novel nSMase2 inhibitors
(A) Western blot analysis of small extracellular vesicle (sEV) fractions prepared from equal amounts of DMSO and DPTIP or PDDC treated HCT116 cells, and probed for the exosomal markers Alix, CD63, and Syntenin. (B) Signal intensity quantification of Alix, CD63, and Syntenin in sEV fractions from (A) by normalizing the signal to loading control GAPDH levels in the corresponding cell lysates in (C) before normalization to their respective control. (C) Western blot of the corresponding cell lysates (CX) from (A), probed for loading control GAPDH in addition to the exosomal markers from (A). (D) Quantifications of intracellular Alix, CD63, and Syntenin signal intensity from (C) normalized to loading control GAPDH before normalization to the respective control. (E) Western blot analysis of Alix, CD63, Syntenin, and CD81 in sEV fractions isolated from DPTIP or PDDC treated HeLa cells. (F) Quantifications of Alix, CD63, Syntenin, and CD81 in sEV fractions from (E) normalized to GAPDH levels in the corresponding cell lysates (G) before normalization to the respective control. (G) Western blot analysis of exosome markers in corresponding cell lysates (CX) from (E). GAPDH was probed as the loading control. (H) Quantifications of intracellular Alix, CD63, Syntenin, and CD81 signal intensity from (G) normalized to loading control GAPDH before normalization to the respective control. (mean ± SD; *P < 0.01, **P < 0,001, ****p < 0.0001; Student t-test; n = 3 independent experiments).
However, in HeLa cells both inhibitors did neither reduce the marker secretion into the supernantant nor led to an intracellular accumulation (Fig. 1E-F). From these experiments we concluded that DPTIP and PDDC can strongly inhibit EV marker secretion into the supernantant in some cell lines, but not in others. We therefore continued our analysis of these inhibitors in HCT116 cells and further evaluated the intracellular effects.
PDDC in endosomal acidification and Sorting of CD63
We next tested whether these inhibitors affected cellular fitness of HCT116 cells. Moderate concentration of GW4869 (5 μM) as well as SMPD3 KD do not impair the viability of different cells analyzed (Choezom & Gross, 2022). Here, cell vialbility was measured by ATP-based luminescense assay after 16 hours of treatment of HCT116 cells and was significantly reduced by 10 μM of DPTIP, but not by 10 μM of PDDC (Fig. 2 A).
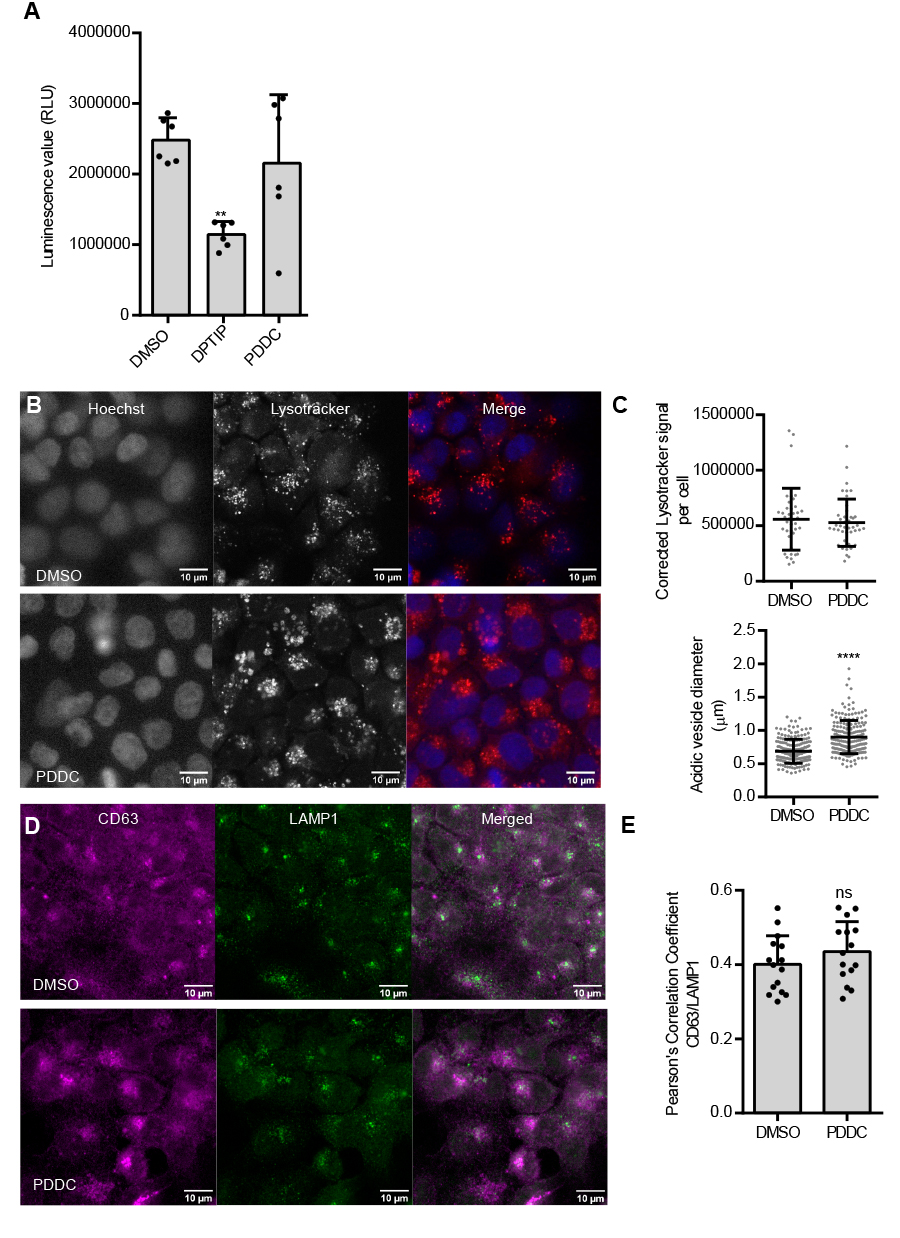
Figure 2: PDDC in endosomal acidification and Sorting of CD63
(A) Viability assay of HCT116 cells treated with DMSO, DPTIP, and PDDC for 16 h. Ordinary one-way Anova followed by Dunnett’s multiple comparisons test; **p < 0.001; n = 3 independent experiments. (B) HCT116 cells treated with DMSO (Ctrl) and 10 μM of PDDC for 16 h were stained with Lysotracker and DAPI and analyzed by confocal microscopy. (C) Quantifications of corrected Lysotracker signal per cell (above) and Lysotracker puncta per cell (Below) from (B). Unpaired Student’s two-tailed t-test. *p < 0.01 ,****p < 0.0001. (D) Confocal microscopy images of HCT116 cells treated with DMSO (Ctrl) and 10 μM of PDDC for 16 h co-stained for exosomal/MVB marker CD63 and lysosomal marker LAMP1. (E) Pearson’s correlation coefficent between CD63 and LAMP1 from (D). (mean ± SD; Unpaired Student’s two-tailed t-test; n = 20 cells).
Previously, we found that GW4869 treatment and SMPD3 knock down increased endosomal acidification, accumulated secretory MVB intracellulaly and impaired exosome secretion (Choezom & Gross, 2022). Therefore, we compared lysotracker staining of acidified endosomal compartments in control and PDDC treated HCT116 cells. Lyostracker signals were comparable but the diameter of lysotracker puncta was significanly increased in PDDC treated cells (Fig. 2 B, C), indicating that acidified compartments are dilated, a sign of impaired endosomal/lysosomal maturation/function (de Araujo et al, 2020).
To check whether trafficking of EV protein CD63 was impaired, we costained CD63 and lysosomal marker LAMP1 in control and PDDC-treated HCT116 cells, Pearson correlation coefficent was similar, but CD63 appeared to acummulate perinuclearly in PDDC cells compared to the diffuse CD63 staining in control cells (Fig.2 D, E). We concluded that PDDC might impair EV secretion, but did not lead to EV marker accumulation because lysosomal degradation seemed to be functional, as EV markers were not accumulating in the cell extract (Fig.1 C, D).
DPTIP and PDDC are inducing basal autophagy
Next, we wanted to investigate whether nSMase2 activity was affecting autophagy in HCT116 cells (Fig. 3).
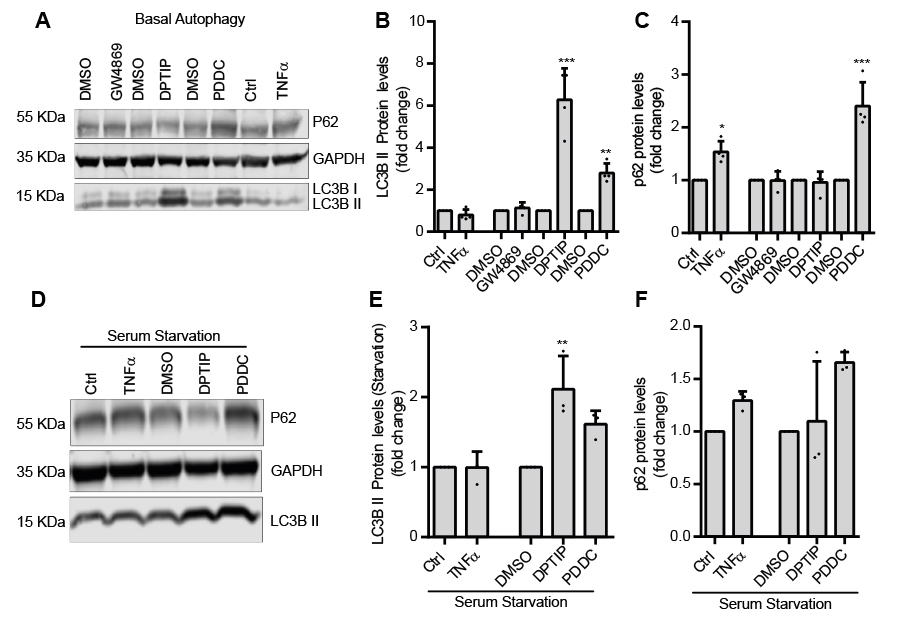
Figure 3: DPTIP and PDDC are inducing basal autophagy
(A) HCT116 cells treated with the indicated drugs for 16 h and lysates were immunoblotted against P62, LC3B-I, LC3B-II, and loading control GAPDH. (B) Quantifications of LC3B-II and P62 protein levels under basal conditions from (A). Unpaired Student’s two-tailed t-test; **p < 0.001, ***p < 0.0009; n = 3 independent experiments. (C) HCT116 cells grown in serum-depleted medium and treated with the indicated drugs for 16 h and lysates were immunoblotted against P62, LC3B-I, LC3B-II, and loading control GAPDH. (D) Quantifications of LC3B-II and P62 protein levels under starved conditions from (C). Unpaired Student’s two-tailed t-test; **p < 0.001; n = 3 independent experiments.
NSMase2 can be induced by stimulating with TNFα (Philipp et al, 2010) and inhibited with both DPTIP and PDDC. Autophagy was monitored by staining for two autophagic markers LC3B lipidation and p62. LC3B lipidation which restults in two bands LC3B-I and II, the lipidated form of LC3B that associates with forming autophagosomes as the first step in autophagic degradation (Mizushima & Yoshimori, 2007). P62 binds ubiquitinylated autophagic cargoes via a C-terminal ubiquitin-binding domain (UBA) and bridges the cargo with lipidated LC3 proteins anchored on the nascent autophagosome membrane (Kehl et al, 2019). In this way, P62 acts as a substrate-delivering-adaptor which is eventually co-degraded with the autophagosomes. Under the basal condition, although TNFα stimulation showed no change in LC3B lipidation levels, nSMmase2 inhibitors DPTIP and PDDC treatment led to a significant increase in the levels of lipidated LC3B (Fig. 3 A,B). These data might indicate increased autophagosome formation upon nSMase2 inhibition with PDDC and DPTIP. In contrast, nSMase2 inhibition with GW4869 showed no effect on the levels of LC3B lipidation. Surprisingly, TNFα and PDDC treatment increased P62 protein levels (Fig. 3 A,C).
We then next monitored lipidated LC3B and P62 levels by inducing autophagy with serum starvation upon nSMase2 activation and inhibition (Fig. 3D-F). DPTIP treatment increased lipidated LC3B levels under serum starvation conditions as well (Fig. 3 D,E). This might indicate that in contrast to GW4869, these novel inhibitors further affect endosomal function by altering the degradation of accumulating EV cargo that cannot be secreted from these cells.
Conclusion
The detailed characterization of EV biogenesis pathways has seen a huge increase in publication in the last 5 years and inhibition of nSMase2 activity is often used to block one of the several pathways of ILV formation. As more and more different EV subpopulations are characterized by content and biogenesis pathway, it becomes clear that cell specificity plays a major role in the effect of nSMase2 inhibition to block EV secretion (neuronal cells, versus cancer cells, versus glandular cells). The tissue of origin could be the major contributor to this, for example in cancer cells or glandular cells with a highly expanded endosomal/trafficking compartment (de Araujo et al, 2020). Another factor influencing the contribution of nSMase2 activity to EV secretion could be the order of endosomal trafficking events in those different cell types. As cancer cells often activate autophagy pathways and are subjected to cellular stress, this could lead to autophagic secretion of these damaged/stress components as a relief mechanism.
A recent publication found that nSMase2 activation serves as a repair mechanism of lysosomal compartments. Ca2+ influx, due to damaged membranes, activates a Ca2+ -dependent flippase and sphingomyelin at the cytoplasmic leaflet is hydrolysed by nSMase2 for intraluminal budding of these damaged membrane stretches (Niekamp et al, 2022).
Taken together, we characterized novel inhibitors of nSMase2, PDDC and DPTIP, for their potential in blocking EV secretion and perturbing EV biogenesis in the endosomal compartment. As lipid composition and the plasticity of the endosomal compartment is very cell type dependent, PDDC and DPTIP seem to have cell type specificity that can be explored for the analysis of EV biogenesis with close monitoring of lysosomal and autophagic degradation pathways.
Material and Methods
Cell culture and Transfection
HeLa (kindly provided by Holger Bastians, Goettingen) and HCT116 (DSMZ) cells were maintained in DMEM (Thermo Scientific Life technologies, 52100021) supplemented with 10 % fetal calf serum (Biochrom) and 10 μg/ml Penicillin/Streptomycin (Sigma Aldrich, P4333) at 37 °C in a humidified atmosphere with 5 % CO2. Cells were routinely checked for mycoplasma contamination and were treated with the following drugs for 16 h: DPTIP (10 μM, Slusher lab,Johns Hopkins University), DPTIP(5 ng/ml, Slusher lab, Johns Hopkins University), TNFα (5 ng/ml, Peprotech, 300-01A).
Antibodies
The following antibodies and concentrations were used for Western blot (WB) or for immunofluorescence staining (IF): Alix (WB 1:1000; Santa Cruz), CD63 (WB 1:100; IF 1:10; Hybridoma), Syntenin (WB 1:1000; IF 1:100; Abcam), GAPDH (WB 1:1000; Millipore), Calnexin (WB 1:1000; IF 1:100; Abcam), LAMP1 (WB 1:1000, Abcam, ab24170 ); Syntenin (WB 1:1000, Abcam, ab133267); P62 (WB 1:1000, Abnova); LC3B (WB 1:1000, Santa Cruz)
Small extracellular vesicles purification
sEVs were purified by differential centrifugation as previously described (Menck et al, 2017). In short, cells were incubated with an sEV-free DMEM for defined periods. EV-free DMEM was obtained by ultracentrifugation of FCS diluted to 40 % in DMEM at 4 °C overnight at 100,000 g. The conditioned medium was collected and subjected to sequential centrifugation steps at 750 g (10 min), 1500 g (10 min), and 14,000 g (30 min), before pelleting sEV at 100,000 g for 1 h. This sEV pellet was termed P100 and was lysed with RIPA lysis buffer diluted at 1:1 with PBS.
Western blot analysis
Cell and sEV lysates, in SDS PAGE sample buffer, were boiled for 5 min before separating the protein on 4 12 % gradient gels (Bolt Bis-Tris Plus Gels, Thermo Scientific). Separated Proteins were then transferred onto PVDF membranes (Merck) and blocked with 5 % (wt/vol) milk-TBST for 30 min. Membranes were then incubated in primary antibody solutions overnight at 4 °C. After washing, membranes were incubated with fluorescently labeled secondary antibodies at room temperature in the dark and protein bands were detected using the Li-COR Odyssey system. Quantitative measurements of the protein bands were done with Fiji/ImageJ (NIH).
Immunostainings, microscopy, and image analysis
Cells were grown in 8-well microscopic coverslips (Sarstedt, 94.6170.802) and treated as indicated. Cells were then fixed and permeabilized with 4 % paraformaldehyde and 0.2 % Triton-X-100. The slides were then blocked in 3 % bovine serum albumin (BSA) diluted in PBS, followed by 90 min incubation with primary antibodies. After washing three times, cells were incubated with secondary antibodies conjugated to Alexa-Fluor-488 or 546. Nuclei and actin were labeled by Hoechst and conjugated-phalloidin respectively. The cells were visualized with a Zeiss LSM780 confocal microscope (Plan Neofluor 63X/oil NA 1.4 objective). Staining and microscopy conditions were kept identical for comparisons. Mean fluorescence intensity of puncta, the number of puncta/cell, and colocalization quantifications were performed using available pipelines with some modifications in CellProfiler (Broad Institute of MIT and Harvard).
Lysotracker staining
were incubated with 200 nM Lysotracker DND-red-99 (Thermo Scientific Life technologies L7528) for 45 min to label acidic compartments and fixed with 4 % paraformaldehyde after washing 3 times with PBS. The cells were visualized with a Zeiss LSM780 confocal microscope (Plan Neofluor 63X/oil NA 1.4 objective). Staining and microscopy conditions were kept identical for comparisons. Mean fluorescence intensity of puncta, the number of puncta/cell, and colocalization quantifications were performed using available pipelines with some modifications in CellProfiler (Broad Institute of MIT and Harvard).
Viability assay
Cell viability was measured by performing a CellTiter-Glo assay (Promega, G8461). Cells were seeded in a 96-well plate. After the indicated treatment, 100 μl of the cell titer glow reagent (Promega) were added to each well (1:1). The plate was incubated on a shaker for 2 min at RT to allow cell lysis and then incubated without shaking for 10 min to allow luminescence signal stabilization. The signal was measured using a luminometer and data were analyzed using MikroWin 2000 lite Version 4.43.
Statistics
All experiments were carried out at least in biological triplicates. Statistical significance was calculated by ONE-WAY ANOVA followed by Dunnett’s multiple comparisons test or unpaired student’s t-test when appropriate.
Acknowledgments
The authors would like to thank AG Bastians (University of Goettingen) for HeLa cells. We thank Dr. Karen Linnenmannstöns, Dr. Leonie Witte, and Dr. Pradhipa Karuna M for critically reading the manuscript and Mona Honemann-Capito for her excellent technical and scientific support.
Author Contribution
D. C.: Conceptualization, Investigation, Formal analysis, Writing – Review & Editing. J. C. G.: Conceptualization, Writing – Original Draft, Review & Editing, Funding acquisition.
Conflict of interest
The authors declare that they have no conflict of interest.