An optimized protocol for the enrichment of small vesicles from murine and non-human primate heart tissue
DOI: https://doi.org/10.47184/tev.2022.01.03Over the last few years, the interest in extracellular vesicles (EVs) function has exponentially grown. However, methods for isolating these small vesicles from tissue are still not trivial. Few protocols that allow EV isolation from whole tissue samples, including the heart, are available and they are based on organ perfusion unsing Langendorff method. In this work, aiming at analysing in vivo biology of small EVs, we implemented a simple method to obtain enrichment of these vesicles from murine heart tissue. We tested a titration of Liberase for tissue digestion, which was subjected to differential ultracentrifugation combined with iodixanol cushion and presented the step-by-step procedure of this protocol. Validation was done with Nanoparticle Tracking Analysis, transmission Electron Microscope and Western Blot analysis of EV markers and organelle contaminants. Furthermore, we tested the suitability of the protocol for isolating EVs from heart tissue obtained from a pre-clinical translational non-human primate animal model. Therefore, this protocol should be suitable for isolating vesicle from human heart tissue. Additionally, this method could potentially be applied beyond heart tissue
Keywords: Tissue, heart, EVs purification, cushioning, murine, non-human primates, EVs quality
Introduction
EVs are a heterogeneous group of bilayer membrane nanoparticles that play roles in diverse intercellular communication in short and long-range distances. Due to their prominence in cellular communication, the study of EVs has recently been gaining great attention from scientists. In the cardiovascular system, physiological and pathological adaptations require a coordinated cell-to-cell communication mediated by cytokines, peptides, hormones and, as recently recognized, also by EVs. Within the EVs group, we can highlight exosomes, which can transport RNA species, lipids, and proteins. These nanoparticles are sensitive to various stress stimuli mediating locally and remotely signaling transduction. Furthermore, they are intensively studied as diagnostic markers as well as cell-free strategies for regenerative medicine [1, 2]. EVs were reported to protect the heart against myocardial infarction and stimulate angiogenesis [3]. Different subtypes of EVs are classified based on physical and/or biochemical properties. In general, based on physical characteristics such as size, EV subtypes can be classified as "small" or "medium/large". Small EVs size ranges between 100 and 200 nm, whereas medium/large EVs size is > 200 nm [9]. Small EVs are released by exocytosis of multivesicular bodies, while whole large EVs and apoptotic vesicles are shed from the plasma membrane. Apoptotic vesicles are in the range of > 1 mm in diameter [4]. EVs, including exosomes (or cardiosomes), were described to be released from all major heart cell types in vitro, suggesting that they play an important role in the cardiovascular system. However, the problem represented by the isolation of these small particles present in the interstitium of cellular tissues in vivo [5], is preventing scientists from advancing more rapidly along this area. Specifically, EVs isolated from heart tissue remained challenging due to the susceptibility of heart cells to hypoxia, including cardiomyocytes, which can cause changes in ultrastructure and secretory activities [6].
The majority of available protocols and data are focused on EVs isolated from cell culture conditioned media or body fluid [4, 5], however, transferability of EV secretion studies in vitro to a disease-dependent condition in vivo is limited [7]. At present, it is still challenging to isolate EVs from tissue homogenates and no standardized protocol has yet been develop that can be considered as a gold standard [4]. Differential centrifugation combined with several steps of further separation including density gradient (sucrose or preferably iodixanol) is recommended for purification of EVs along with co-pelleted protein complexes and lipoproteins [8]. EVs can be isolated from hearts via Langendorff perfusion however, the buffers used can cause exosome-sized and calcium-phosphate nanoparticle formation, due to the spontaneous formation in Ca+2- containing bicarbonate buffer. These features, can directly interfere with some analysis such as nanoparticle tracking analysis [4, 9].
In this study, we aimed to obtain interstitial EVs contained in the heart in vivo and reflecting the physiology of the murine heart with an easy-following protocol with gentle digestion, as an alternative to Langendorff perfusion. Standard methods of EV characterization including, transmission electron microscopy (TEM), nanoparticle tracking analysis (NTA) and detection of positive and negative markers for EVs were used to characterize the enriched EVs. EVs were reported to be produced by almost all organisms and cell types studied; however, research has mainly focused on EVs of human or mouse origin, leaving other species less investigated [10]. In this article, we showed the suitability of the protocol for isolating EVs from heart samples of non-human primates (Callithrix jacchus, common marmoset); a pre-clinical animal model providing the optimal compromise for human related therapeutic studies due to the high homology with the human genome [11]. Currently, only physical characterization of Callithrix jacchus derived cardiac EVs can be performed due to the lack of suitable specie-specific antibodies, which remain to be established.
Methods and Materials
Solution preparation
Solutions are provided in Table 1, 2 and 3.
Table 1: Normal Tyrode's Solution pH 7.35
Ingredient | Molar mass [g/mol] | Final | For 1 l | Manufacturer | Order number |
---|---|---|---|---|---|
NaCl | 58.44 | 150 mM | 8.766 g | Roth | 9265.2 |
HEPES | 238.3 | 5 mM | 1.2 g | Roth | HN77.5 |
KCl | 74.6 | 5.4 mM | 0.4 g | Roth | P017.1 |
Glucose | 180.16 | 10 mM | 1.802 g | AppliChem | A3666 |
Na-Pyruvate 100mM | 58.44 | 2 mM | 20 ml | Gibco | 11360070 |
MgCl2 x 6 H2O | 246.5 | 1.2 mM | 0.24 g | Sigma Aldrich | M2393 |
ddH2O | - | - | add to 1 l | - | - |
Table 2: Perfusion Buffer
Ingredient | Molar mass [g/mol] | Final | For 1 l | Manufacturer | Order number |
---|---|---|---|---|---|
Taurin | 125.1 | 10 mM | 1.25 g | Roth | 4721.2 |
2,3-Butanedione monoxime | 101.1 | 12.38 mM | 1.25 g | Sigma Aldrich | B0753 |
Normal Tyrodes | - | - | add to 1 l | - | - |
Table 3: Digestion Buffer
Ingredient | Molar mass [g/mol] | Final | Manufacturer | Order number |
---|---|---|---|---|
Liberase DH | 5 mg/ml | 1 mg/ml 2 mg/ml 3 mg/ml | Roche | 5401059001 |
DNAse I | 100000 UI/ml |
| Roche | 04716728001 |
Perfusion Buffer | - | add to final volume ml | - | - |
Perfusion Buffer is prepared fresh and adjusted to pH (7.35 – 7.4). The incubator pre-warmed at 37 °C containing the shaking device. Collagenase D and DNase I are thawed by leaving the required aliquots on ice.
OptiPrep for iodixanol cushion
Solutions of 30 % and 10 % iodixanol were prepared in a15 ml tube according to the following recipes:
30 % iodix. Solution = 4 ml HM + 6 ml 50 % iodixanol stock solution
10 % iodix. Solution = 8 ml HM + 2 ml 50 % iodixanol stock solution.
Filter iodixanol solutions with 0.1 µm filters and store at 4 °C until use.
The preparations of Working Solution (WS) 1 and Homogenization Medium are provided in Tables 4 and 5.
Table 4: Working Solution 1
| Concentration | Volume for 50 ml |
---|---|---|
Sucrose | 2.5 M | 5 ml |
EDTA | 0.5 M | 0.6 ml |
Tris | 1 M | 3 ml |
H20 |
| 41.4 ml |
Table 5: Homogeneization Medium
| Concentration | Volume for 50 ml |
---|---|---|
Sucrose | 2.5 M | 5 ml |
EDTA | 0.5 M | 0.1 ml |
Tris | 1 M | 0.5 ml |
H20 |
| 44.5 ml |
For preparing 45 ml of Working solution 2 it is necessary to mix 41.7 ml of OptiPrep and 3.3 of WS1.
Heart preparation
The Niedersachsen animal review board (AZ-G 20.3434 and 20.3458, mice and marmoset, respectively) approved all animal experiments. Hearts were extracted from six-months old C57BL/6 mice under anaesthesia with isoflurane and euthanized by cervical dislocation. Pieces of marmoset hearts were obtained in frame of other studies. Isolated heart tissues were weighted and immediately transferred to a 10 cm Petri dish filled with PBS. Blood was removed and ventricles were dissected. The hearts were kept on ice during the time between removing and processing the heart.
Tissue dissociation
Hearts were transferred to a 10 cm Petri dish filled with 1 ml Perfusion Buffer and then gently sliced using a sterile disposable scalpel. Atria and vessels were removed and tissue was dissociated until all pieces had a homogeneous size. Each heart was processed separately.
Enzymatic digestion
Using a cut 1 ml tip attached to a P1000 pipette, the tissue pieces were transferred to a 2 ml polypropylene tube containing 1.5 ml of Perfusion Buffer with a mixture of the corresponding concentration of Collagenase D (3 mg/ml, 2 mg/ml or 1 mg/ml) and DNAseI when corresponding. The 2 ml tube containing tissue pieces with enzymes were immediately incubated at 37 °C for 30 min. Then, the lysate was transferred to a fresh 15 ml polypropylene tube using a 1 ml tip, and afterwards homogenized by pipetting up and down. The tube was inverted and the tissue pieces were allowed to settle for 1 min.
Tissue filtering and optional storing
Supernatant was filtered through a 70 μm cell strainer into a 50 ml fresh polypropylene tube. After that, samples were stored at -20 °C until further use.
Differential centrifugation and ultracentrifugation
Samples were thawed and centrifuged at 300 g for 10 min at 4 °C in a Thermo scientific Heraeus Fresco21 centrifuge. The supernatant was carefully transferred to a new 2 ml polypropylene tube. This was carried out very cautiously so as not to disturb the pellet during the supernatant transfer. After that, the process was repeated again at 2000 g for 20 min at 4 °C and at 16500 g for 20 min at 4 °C. Then, the supernatant was carefully transferred to a 2 ml Open-Top Thickwall Polycarbonate Tube (#343778) and filled up with PBS. Tubes were balanced within 100 mg precision, placed in a ml A-130 (#367114) and centrifuged at 118000 g for 2.5 h at 4 °C. Acceleration and deceleration was set to the maximum rate and the ultracentrifuge (UC) immediately started. Supernatant was removed and the pellet was resuspended in 600 μl PBS. Samples were stored at 4 °C overnight.
Iodixanol cushion
Inside a laminar flow hood, 1.5 ml of 60 % (wt./vol) OptiPrep was transferred to the bottom of a 6 ml Open-Top Thinwall Polypropylene Tube (#326819) and thoroughly mixed with 500 μl sample using a 1 ml syringe. The tube was tilted slowly to 60° and 2 ml of 30 % (wt/vol) iodixanol solution was transferred drop by drop, leaving the drops to lie down on top of the previous layer by sliding along the tube wall. Again, the tube was slowly tilted to 60° and transferred with 1.5 ml of 10 % (wt/vol) iodixanol solution drop by drop, leaving the drops to lie down on top of the previous layer by sliding along the tube wall. A mock control tube was prepared which contained only PBS. The tubes were labelled at the border of each layer. The tubes were balanced with 10 mg precision and placed into a ml S-50 rotor (#367280) and centrifuged at 186000 g for 2.5 h at 4 °C. Acceleration and deceleration were set at the maximum rate. Afterwards, 1.5 ml of the upper layer were removed and discarded and the following 500 μl were transferred into a new 6 ml polypropylene centrifuge tube (Company; #326819).
PBS washing
The 6 ml polypropylene centrifuge tube was filled with PBS, balanced with 100 mg precision, placed into a ml S-50 rotor and centrifuged at 118000 g for 1.5 h at 4 °C. Acceleration and deceleration was set to the maximum rate and the ultracentrifuge immediately started. Supernatant was removed and the precipitate resuspended in 100 μl PBS for further processing.
Transmission Electron Microscope (TEM)
TEM grids (copper, 150 hexagonal mesh, Science Services, Munich, Germany) were coated with a formvar film, put on top of 10 μl droplets of the exosome fraction and incubated for 10 min. Then, grids were washed 5 times with PBS followed by an incubation on droplets of water. For contrasting, grids were stained for 5 min on droplets of uranylacetate-oxalate and followed by 5 min incubation on droplets of a 1:9 dilution of 4 % uranylacetate in 2 % methylcellulose. These solutions were prepared as described [11]. After blotting the methylcellulose from the grids using a filter paper and drying of the methylcellulose film, samples were imaged with a LEO912 transmission electron microscope (Carl Zeiss Microscopy, Oberkochen, Germany) and images were taken using an on-axis 2k CCD camera (TRS, Moorenweis, Germany).
Nanoparticle Tracking Analysis (NTA)
The size distribution of the EVs was analysed using a Malvern Panalytical NS300 instrument equipped with nanoparticle particle tracking software (Version NTA 2.3 Analytical Software). According to the manufacturer’s recommendation, the samples were illuminated by a laser at 488 nm (Blue 488), and the movement of nanoparticles due to Brownian motion was recorded for 60 s at a mean frame rate of 20 frames per second. Each process was repeated three times. EV particles were diluted in PBS (1:50). Camera level 14, screen gain 10.8, detection threshold 5. For each sample, a total of five videos of 30-60 s were used to measure the nanoparticle concentration per sample. The videos were analysed by the NanoSight NTA and the concentration of particles, size distribution, and the general mean and mode of the samples were obtained as average of the five measurements.
Western Blot Analysis (WB)
Proteins were extracted from tissue by the addition of lysis buffer (10 mM Tris/HCl pH 7.5; 150 mM NaCl; 0.5 mM EDTA; 0.5 % NP-40) containing proteases and phosphatases inhibitor cocktail (Roche). After clarification (12000 g at 4 °C, 20 min), protein concentration was quantified with Bradford assay and the different samples were resuspended in sample buffer. For EVs, 14 μl of final preparation (per heart) was lysed adding 6 μl of 4X Laemmli buffer and boiled 5 min at 95 °C. Samples were loaded on TGX-stain free blots gradient gel from Bio-Rad. Gel were run at constant 250 V, followed by semi-dry transfer. After blocking (3 % BSA in TBST) the membranes were incubated with primary antibodies overnight (Table 6).
Table 6: Antibodies
Name | Manufacturer | Species | Dilution | Order number |
---|---|---|---|---|
anti-CD81 | abcam | rabbit | 1:1000 (WB) | EPR21916 |
anti-TSG101 | abcam | rabbit | 1:1000 (WB) | EPR7130(B) |
Anti-Calnexin | abcam | rabbit | 1:1000 (WB) | EPR3633(2) |
Anti-rabbit IgG, HRP-linked Antibody | Cell signaling technology | rabbit | 1:5000 (WB) | 7074 |
Anti-mouse IgG, HRP-linked Antibody | Cell signaling technology | mouse | 1:5000 (WB) | 7076 |
Membranes were incubated in secondary antibody for 1 h at room temperature (anti-mouse IgG (H+L) HRP conjugate (Bio-Rad) 1:5,000), developed (ECL Amersham) and imaged using ChemiDoc Touch Imaging System (Bio-Rad).
Protocol step-by-step
Liberase titration for EVs enrichment
Mouse hearts were extracted and the EV isolation protocol was performed using digestion conditions in Table 7 in order to test optimal preparations.
Table 7: Antibodies
Condition | mg of tissue | Liberase concentraction (µg/ml) | 40 U DNAse I |
---|---|---|---|
1 | 0.36 | 3 | - |
2 | 0.24 | 3 | + |
3 | 0.32 | 2 | - |
4 | 0.37 | 2 | + |
5 | 0.20 | 1 | - |
6 | 0.25 | 1 | + |
Liberase enzyme is a mixtures of highly purified collagenase and neutral protease enzymes, formulated for efficient, gentle, and reproducible dissociation of tissues from a wide variety of sources (Roche Diagnostics GmbH, Mannheim, Germany) [12] and it was used in the present study. Samples were processed as indicated in Figure 1.
The samples were frozen and the experiment proceeded the following day. After thawing samples, differential centrifugation was performed and each precipitate was stored in -20 °C. Afterwards, samples were ultracentrifuged and a “reddish” pellet was observed (Figure 2A). T
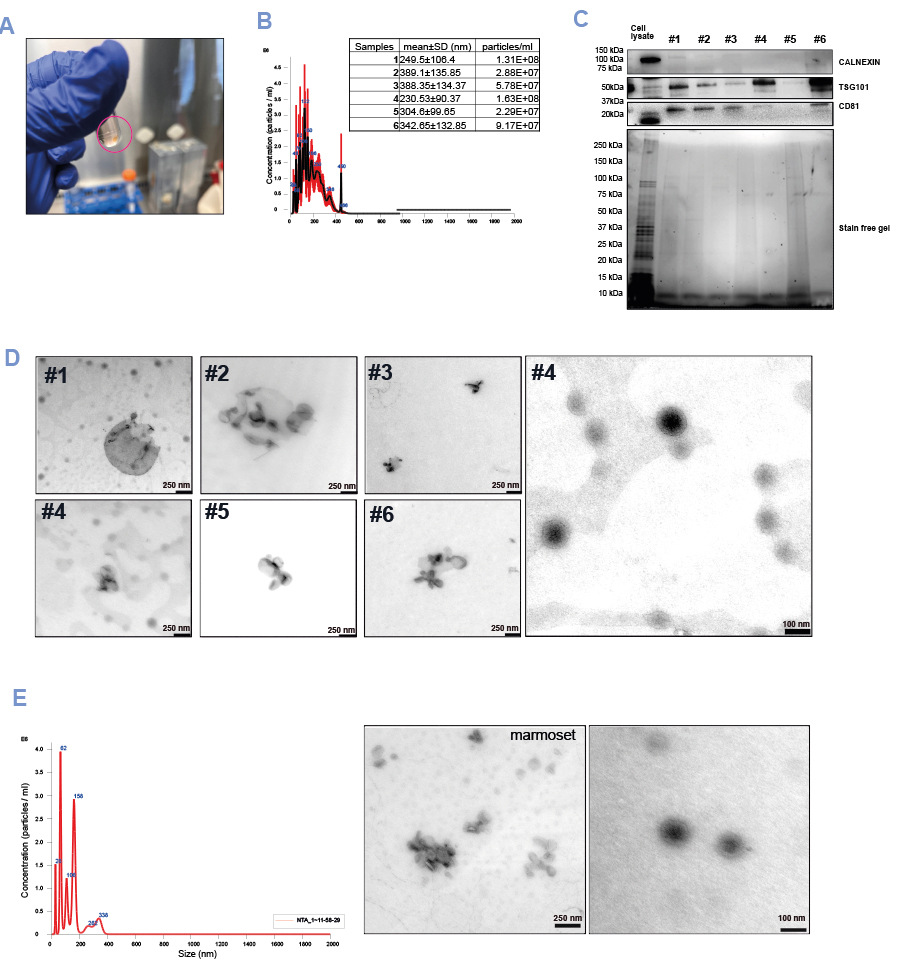
Figure 2: A. “Reddish” pellet observed after differential centrifugation including ultracentrifugation. B. Representative NTA examples for sample #4 as a mean of five consecutive measurements confirming isolation of EVs < 250 nm in diameter and average (avg) of 8.25E+07 particles/ml per isolated heart that was subjected to EV isolation. C. Western blot showing expression of the exosome transmembrane CD81 and cytosolic TSG101 protein markers as well as the endoplasmic reticulum marker Calnexin. D. Electron microscopy confirmed the isolation of round and cup-shaped EVs vesicles with 50-300 nm in all the conditions in murine hearts. Specifically, condition #4 showed more concentrated and pure vesicles <250 nm. E. Electron microscopy confirmed the isolation of small EVs (<250 nm) particles in marmoset heart tissue. Scale bar: 250 and 100 μm.
This pellet was resuspended in 500 μL PBS and kept in the fridge until the following day. From the 500µL resuspended pellet, 10 μl were taken in order to have a 1:50 dilution (PBS), 500 μL final volume. The rest of the sample was ultracentrifuged using an iodixanol cushion. After this stage, the upper layer was removed (10 % iodixanol) and the liquid corresponding to the “white ring” (~500 μl) that in some occasions was observed in the intersection between 10 % and 30 % layers was kept. These 500 μl were then washed with PBS by UC and the pellet was afterwards resuspended in PBS.
Sample analysis
The final EV preparation obtained by performing the protocol described in Figure 1, was analysed by particle size determination using NTA, which showed a mean size of purified EVs was 230.54 ± 90.37 nm and an average of 8.18e+07 particles/ml per isolated heart among conditions (Figure 2B). Further validating the fraction obtained by UC and iodixanol cushion, Western blot analysis demonstrated an enrichment of the EV marker protein TSG101 and CD81 in fractions #1-4 and #6, compared to cell lysate. In contrast, expression of Calnexin, which generally represents ER membrane contamination [9], was completely absent in the fractions #4-5, while traces of expression were observed in the other fractions (Figure 2C). Total protein contained in the EVs was measured showing an average concentration of 587.5 mg/ml per murine heart. These fractions were further characterized by TEM analysis, which showed vesicles with uniformly round and cup-shaped morphology with a 50- to 300-nm diameter. Specifically, condition #4 showed the best enrichment of small EVs (Figure 2D). Together, the biochemical and physical characterization of different tissue digestion conditions showed that condition #4 (2 μg/ml with DNase I) delivered the most robust enrichment of EVs from murine adult heart tissue, with a clear EV-protein expressing fraction (TSG101 and CD81) and absence of contaminant (calnexin) as well as a small and medium EVs demonstrated by NTA and TEM. The isolation protocol with condition #4 was confirmed with further isolations. This data further validated the EV isolation protocol as well as characterized these particles as small and medium EVs based on the biochemical and physical characterization.
Application of the method for other species
This optimized protocol was further applied for isolating EVs from heart tissue of non-human primates Callithrix jacchus (common marmoset), which provides an optimal model for human related therapeutic studies [10]. Liberase treatment was adjusted for heart tissue of marmoset (0.5 mg/ml). The same procedure as explained in Figure 1 was applied and the preparation was subjected to NTA and TEM analysis (Figure 2E). An average of 1.08E+08 particles/ml was obtained. Due to the lack of specific antibodies for Callithrix jacchus, Western blot analysis could not be performed and are currently under development. However, the physical characterization indicated isolation of a similar EV-fraction as obtained and characterized for the mouse heart.
Conclusion
The methods focusing on isolation of tissue-derived EVs are rather limited, hence protocols that are optimized for isolation of EV subpopulations from tissues are still under continuous development [13]. Specifically from the heart, EVs were isolated by perfusion using the Langendorff technique [3]. This technique requires time, a dedicated apparatus and training. Moreover, it may lead to the generation of exosome-sized particles due to the use of Ca2+containing bicarbonate buffer, which can interfere with the detection of true EVs [3, 14]. EVs isolated from heart tissue remained challenging due to the susceptibility of heart cells to cause changes in secretory activities and activate stress under hypoxic conditions [5]. Thus, we aimed to establish a simple method for the isolation of EVs from heart tissue. The protocol tested and validated in this study, allows for EV enrichment in less than 8 hours, which can be split in two days if necessary. Importantly, this protocol does not require previous specialized skills in the lab other than basic knowledge of molecular biology as well as specialized equipment other than an UC, which is commonly equipped in every lab.
Our protocol is based on a gentle enzymatic dissociation of tissue using Liberase, which favours the separation of EVs from cells and extracellular matrix, which in turn leads to less cell disruption [12]. Since a limitation of UC is the co-pelleted protein complexes and lipoproteins, a density gradient for further separation is recommended (sucrose or preferably iodixanol) [15]. Thus, our protocol includes a combination of differential centrifugation steps and an iodixanol density gradient (OptiPrep). However, no EV isolation method that can be considered gold standard for tissue has been yet developed and absolute purification of EVs from other particles is an unrealistic goal [9]. Thus, it is reasonable to speak about separation, concentration or enrichment of EVs. The present method is based on previous protocols for EV isolation from melanoma metastatic tissues [13]. This allows interstitial EVs to be separated from the pieces of tissue in which they are contained.
In order to define a protocol with a good separation of EVs from other non-EV components we tested different digestion conditions. All conditions showed a considerable amount of particles and protein concentration that was achieved, however the purity indicated by lack of ER contaminant markers as well as the concentration of the EVs as demonstrated by TEM were exhibited in the condition with 2 μg/ml with DNase I containing buffer; identified as the most robust EV separation. Enzymatic treatment of the tissue pieces is critical for successfully harvesting significant amount of EVs, based on the cellular complexity of the starting tissue material and the presence of EVs in the extracellular matrix of these tissues [13]. Although we cannot completely exclude the presence of other cellular components or intracellular vesicles, our protocol exhibited significant enrichment of EVs and indicated that sequential UC and further separation with an iodixanol gradient, allowed for enrichment and concentration of EVs. In line with the MISEV2018´s guidelines, we have shown that our protocol yields EVs with physical and biochemical characteristics of small EV or exosome based on the expression of proteins within the category 1 (CD81, non-tissue specific transmembrane protein) and 2 (TSG101, cytosolic protein) of MISEV2018´s guidelines, marking EVs/exosomes [9].
We have demonstrated the use of the present protocol for EV enrichment from mouse and non-human primate heart. The latter lack biochemical characterization due to the missing species specific antibodies, however the physical characterization of the enriched fractions reflects the isolation in the murine heart. The application of this protocol to other tissues, upon adjustment of Liberase concentration may be suitable. Therefore, we propose this protocol as a useful approach for tissue preparations and vesicle enrichment for murine and non-human primate heart tissue using differential UC, which has long been regarded as a reliable technique. In conclusion, our protocol validated a simple way to enrich EVs by using a combination of different UC steps and density gradients to isolate small EVs, which has been validated on two different types of cardiac tissue.
Acknowledgements
We thank Petra Tuchola (Institute of Pharmacology and Toxicology) for assisting with mouse handling as well as Dr. Zoltán V. Varga and Dr. Zoltán Giricz (HCEMM-SU Cardiometabolic Immunology Research Group, Department of Pharmacology and Pharmacotherapy, Semmelweis University, Budapest, Hungary, Pharmahungary Group, Budapest, Hungary) for assisting with protocol establishment. We thank Boguslawa Sadowski for the TEM and Dr Zuzana Ditte for NTA (Max Planck Institute for Multidisciplinary Sciences). We acknowledge support by the Open Access Publication Funds of the Göttingen University. This work was supported by the DFG grant SFB1002 C07 to LCZ; and INF project to Sara Nußbeck and LCZ; the DZHK (German Center for Cardiovascular Research), and the CRYSTAL3.