Extracellular vesicles – upcoming biomarkers in Parkinson's disease's biofluids
DOI: https://doi.org/10.47184/tev.2022.01.06The search of a biomarker for an early detection of neurodegenerative diseases is one of the biggest challenges of our times. The second most common neurodegenerative disorder Parkinson's disease (PD) is characterized by misfolded alpha-synuclein (a-syn) aggregates within the central nervous system (CNS). Currently, definitive PD diagnosis still requires post-mortem brain examination. As a result, the misdiagnosis of PD based only on clinical symptoms and delayed diagnosis in advanced stages cannot be excluded. Since a-syn aggregates abnormally, it might be an interesting candidate for a biomarker for PD. Lately, extracellular vesicles (EVs) have emerged as potential biomarker in biofluids since accumulating evidence suggests that their content reflects the pathophysiological alterations occurring in their host cells. Interestingly, EVs can cross the blood-brain barrier (BBB) and thus carry information from the CNS to the periphery and vice versa. EVs seem to play a role in other neurodegenerative disorders such as Alzheimer's and prion disease, where they have also shown certain diagnostic potential. For instance, EV isolation protocols have been described to isolate brain-derived EVs from blood samples, increasing their biomarker potential in neurodegenerative disorders. The results published for PD to date are promising: pathology-associated a-syn forms are found in blood-derived EVs, although the underlying mechanisms of formation and release of a-syn-loaded EVs remain unknown. Interestingly, a-syn level correlate with the disease stage, which underlines the importance of neuronal EVs in disease monitoring. Further research extends to other biofluids, like urine, saliva, and cerebrospinal fluid, where EVs can also be found, opening multiple opportunities for more reliable PD diagnosis.
Keywords: Parkinson’s disease, neuronal extracellular vesicles, blood biomarker, neurodegenerative disorders
Parkinson's Disease
Parkinson´s disease (PD), the second most common neurodegenerative disease, is characterized by progressive degeneration of dopaminergic neurons in the central nervous system (CNS), most prominent within the Substantia nigra pars compacta (SNpC). Neuropathologically, PD is defined by the accumulation of misfolded alpha-synuclein (a-syn) as insoluble "Lewy-Bodies", first described by Dr. Friedrich Lewy in 1912 [1, 2]. a-Syn is expressed mainly at presynaptic terminals, however, to date, its function is not entirely understood. It has been shown to be involved in neurotransmitter release and synaptic interaction via the soluble N-ethylmaleimide-sensitive factor attachment receptor (SNARE) complex. In PD, a-syn forms insoluble aggregates and intracellular occlusions, causing cytotoxic damage by mitochondrial, lysosomal, and proteasomal dysfunction, damaged membranes and altered synaptic functions, subsequently leading to neurodegeneration [3]. The toxicity of misfolded oligomer a-syn was so far shown in vivo and in vitro [4, 5].
In patients, PD manifests with cardinal motor symptoms such as resting tremor, rigidity, bradykinesia, and loss of postural reflexes [6], but patients also often suffer from gastrointestinal (GI) symptoms accompanied by altered gut microbiota composition [7]. Interestingly, a-syn aggregates are also found in the GI tract, and recent findings in the field indicate that this misfolded a-syn may originate from the Enteric Nervous System (ENS) [8]. It was postulated that a-syn pathology might start in the gut reaching the brain via retrograde axonal transport through the vagal nerve (Figure 1).
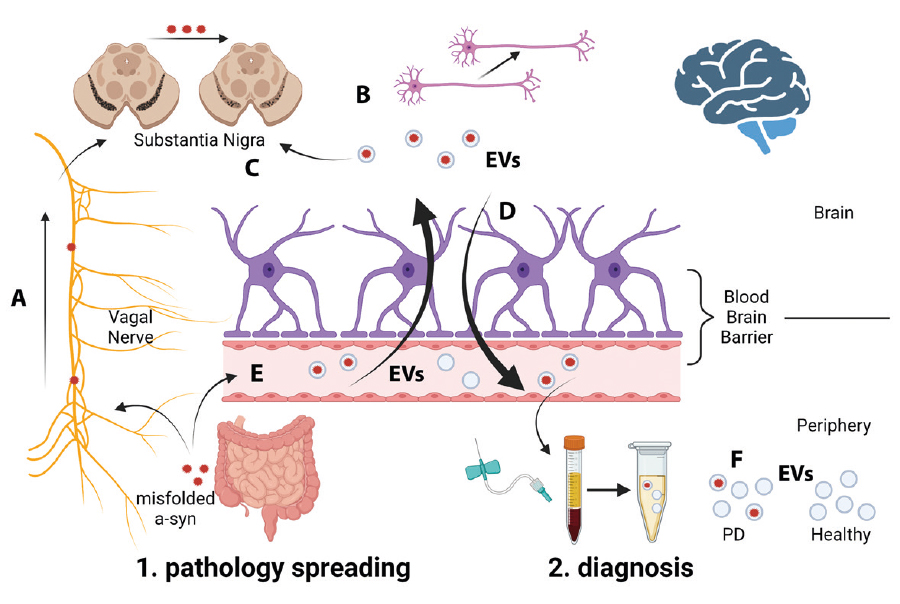
Figure 1: Role and function of blood-derived EVs in PD patients. As EVs are able to cross the BBB, research in the field of neurodegenerative disorders is concentrated on two main aspects: The first is the description of the pathology (1), as EVs in the blood can serve as alternative transport mechanisms of pathologic a-syn aggregates besides the trafficking along the vagal nerve that connects the intestine with the CNS (A). Furthermore, EV-derived a-syn might accelerate spreading intercellularly and through different brain regions (B). In PD, the accumulation of pathologic a-syn aggregates in the Substantia Nigra is linked to the degeneration of dopaminergic neurons (C). Moreover, EVs could also give information about the pathophysiological state of the brain and might be useful for the monitoring of disease state. The second aspect of EVs, is their potential to serve as diagnostic tools (2): Within the blood EVs derive from the brain (D) and other peripheral organs like the intestine (E) and might carry pathologic forms of a-syn (F), which could serve as biomarkers. Figure created with biorender.com
In rat models, a-syn inclusions were detected in the dorsal motor nucleus of the vagal nerve 12h after injection of human brain lysate of PD patients into the animals' intestinal wall [9]. Recently, another mechanism of pathological a-syn spreading has been suggested, namely via extracellular vesicles (EVs) in the bloodstream, which will be further discussed below [10].
Up to this date, a definite diagnosis of PD is only possible after post-mortem autopsy by histopathological detection of aggregated forms of a-syn appearing as Lewy-Bodies in the CNS, especially in the SNpC. Clinical symptoms often overlap with those in other synucleinopathies, like multiple system atrophy (MSA) and dementia with Lewy bodies. This missing biochemical marker results in the fact that around 25 % of clinically diagnosed PD cases are not validated by autopsy afterward. In addition to this high rate of misdiagnosis, there seems to be a long prodromal period between the onset of neurodegeneration and the appearance of the first clinical symptoms. Consequently, most of the dopaminergic neurons (~70 %) in the SNpC have already been lost at the moment of diagnosis, and patients are already highly affected [11]. This poses a significant problem for upcoming therapeutic approaches and clinical interventions. To summarize, the need for reliable and accessible biomarkers is urgent for PD, especially to diagnose the disease in the prodromal phase (before significant damage has occurred to the brain), as an early treatment increases the chances of being curative.
Extracellular vesicles - small entities with high potential
According to the International Society for Extracellular Vesicles (ISEV), EVs are defined as particles released from cells that possess a lipid bilayer and are incapable of replication [12]. Their size range is from 30 to more than 1000 nm, and are able to carry DNA, mRNA, receptors, enzymes, metabolites, and several types of lipids. Due to their cargo, EVs play a relevant role in various physiological but also pathological mechanisms: They are regulators of intercellular communication and could play a cardinal role in cancer development, immune responses, and disease spreading. Nonetheless, since composition of these vesicles is dependent on their origin cells, EVs have emerged as a potential source of biomarkers for various diseases [13].
EVs can be distinguished by physical characteristics, including size or density, biochemical composition, or subcellular origin. The biosynthesis differs between two main subtypes of EVs: the exosomes, which are the product of the endosomal system after the fusion of multivesicular bodies with the cell surface, and the microvesicles, also called ectosomes, that are produced by budding of the plasma membrane [14].
For assay and quality control, EVs should be characterized using at least three different approaches to demonstrate the quality and purity of the samples [15]. First, a physical analysis by microscopy is performed, usually by Transmission Electron Microscopy (TEM) [16]. Secondly, particle size and number can be measured by light scattering technologies, such as nanoparticle tracking analysis and flow cytometry, among other similar techniques [17]. Finally, the biochemical description, e. g. via immunoblotting or ELISA, of at least three different EV-related markers such as tetraspanins CD63, CD81 and CD9 as well as the absence of non-EV markers [18].
For the wide research field of neurodegenerative diseases, specific proteins expressed by brain-derived EVs are essential to separate these from the total EV pool in different biofluids. EV surface proteins can be used for purification of respective EVs for instance by pulldown utilizing specific antibodies. In plasma-derived EVs, the L1 cell adhesion molecule (L1CAM) has been established to purify neuron-derived EVs (NEs) due to its expression in neuronal cells [19-21]. However, there is a vigorous discussion in the field about how reliable L1CAM is as a neuronal marker on EVs since its specificity for CNS-derived EVs is debated. L1CAM shows expression mainly in the CNS, the peripheral nervous system (PNS), but it is also expressed in the distal renal tubules and by endothelial cells [22] (Human Protein Atlas, www.proteinatlas.org). Moreover, L1CAM is also expressed in soluble forms in the blood, therefore, not all the L1CAM found in this fluid would be associated with EVs [22, 23]. On the other hand, another study suggests the neuronal origin of L1CAM-positive EVs, since their proteomic analysis shows an enrichment of CNS proteins like phosphorylated tau (p-tau), neuron-specific enolase (NSE), microtubule-associated protein 2 and neurofilament light chain (NfL). These findings strengthen the notion of L1CAM-positive EVs being predominantly of CNS origin [24]. However, until further analyses, L1CAM-precipitated EVs should be referred to as putatively CNS-derived [25]. The L1CAM approach was useful for obtaining the first results on CNS-derived EVs from blood. In the future, more precise markers to isolate EVs from other brain cell types such as astrocytes, microglia, and oligodendrocytes, also relevant in PD pathology, are needed to receive highly reproducible and confident results. In this regard, NCAM1 has been suggested as a promising marker for neuron-derived EVs, but more work has to be done for validation [26].
New hope in PD diagnosis?
In the case of neurodegenerative disorders, biomarkers in biofluids have a substantial practical value for their easy clinical availability (Figure 2).
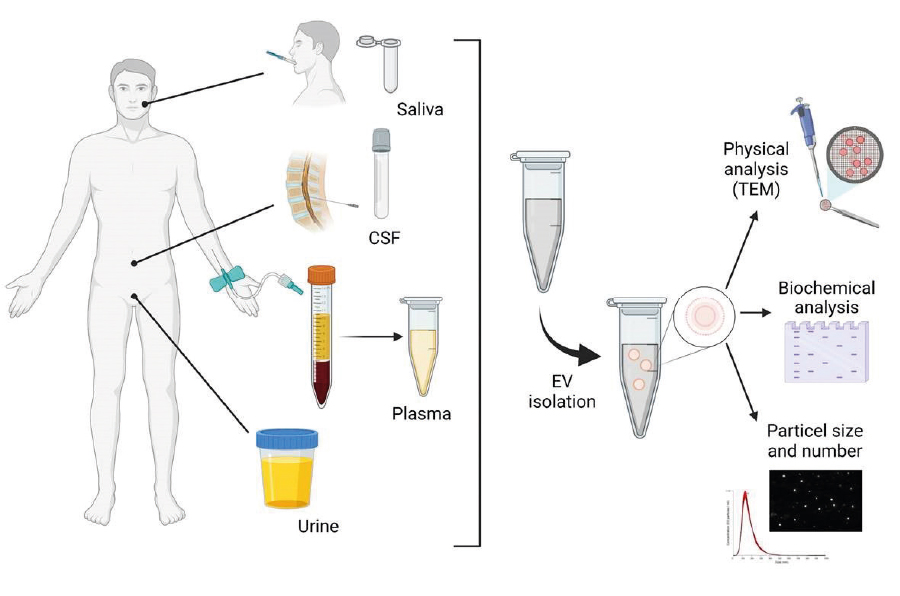
Figure 2: Isolation of EVs from different biofluids. EV isolation protocols exist for different biofluids such as plasma, CSF, saliva, and urine. Those biofluids all have a prominent role in the clinical routine and could be of use for diagnosis of PD. Quality control of EV purification should be validated by biophysical analysis (for example, TEM), biochemical analysis for specific positive and negative markers, and particle size and number description by light scattering technologies. Figure created with biorender.com
For obvious reasons, the biopsy of the brain tissue is impracticable for routine disease screenings. However, there are also limitations, as brain-derived biomarkers can only be found in low concentrations in the blood due to the BBB selective permeability. Furthermore, most biomarkers also show expression in non-neuronal tissues, further complicating their use for clinical diagnostics. In addition, plasma proteases could potentially degrade brain-derived proteins during the sampling process [27]. An ideal biomarker should show a strict relation to the disease progression or pathophysiology, does not need invasive intervention in patients, and can be detected with high sensitivity and specificity, and recent results suggest that in PD EVs could meet those requirements [28].
Several studies regarding neurodegenerative disorders aim to evaluate the potential of EVs in different biofluids as biomarkers [29]. Among these are cerebrospinal fluid (CSF), which is uncomfortable for patients to collect compared to other easily retrievable biofluids like blood, urine, or saliva. EVs are present in all of these biofluids, and their suitability for EV-dependent biomarkers in PD is discussed below (Figure 2). It is important to mention that EVs can cross the BBB in both directions [30] enabling them to function as potential shuttles from the brain into the periphery and vice-versa. The BBB separates the brain from peripheral tissues, including those relevant to the pathogenesis of PD. This boundary plays a vital role in maintaining brain homeostasis and protection from potentially hazardous substances or immune cells in the blood [30]. Findings of increased BBB permeability in PD patients [31] further strengthen the notion of EVs serving as potential biomarkers for the disease [32].
Although EVs might play an important role as biomarkers, it is essential to mention that EVs could also be crucial in the pathogenesis of PD, and neurodegenerative disorders in general, regarding disease-spreading mechanisms across different brain regions and from peripheral tissue, like the intestine to the brain and vice versa (Figure 1). Therefore, isolating EVs from biofluids is also a relevant tool for studying PD pathology [33]. For example, it was shown that PD patient-derived EVs from the CSF incorporate a-syn, which can serve as a seed and transmit its pathologic conformation to monomeric a-syn and thus spread and accelerate a-syn oligomerization [34]. This idea is supported by the fact that EV-associated uptake of a-syn oligomers by recipient cells is more efficient and, interestingly, has a more toxic effect on these cells when compared to EVs free of recombinant a-syn oligomers [35]. Furthermore, EVs showed spreading of pathological a-syn inside the brain among cells and brain regions [36]. Especially the hierarchical distribution of a-syn pathology in the brain between areas is a characteristic hallmark of PD. Further evidence shows that EVs loaded with a-syn can have cytotoxic effects on receiving cells, which implies an important role of EVs on the pathology of PD [37-39]. Another mechanism observed in PD is the removal of extracellular a-syn via endocytosis by astrocytes and microglia to protect neurons from toxic a-syn accumulation. This uptake of a-syn in astrocytes and microglia can cause glial inclusions and induction of inflammation [39].
Blood
Total a-syn levels in the blood have not shown to be a reliable biomarker because of the highly variable release of a-syn from peripheral cells [32] and its predominant occurrence in red blood cells [40]. As recent studies suggest, blood-derived EVs seem to be suitable for analyzing pathological a-syn [32, 41-44].
In mouse models, Shi et al. [32] showed for the first time that radiolabelled a-syn, which was injected into the mouse brain, was shifted rapidly to the periphery and was then detected in blood-derived EVs, purified after L1CAM-pulldown [32]. L1CAM is not expressed in red blood cells and platelets [32], which are the primary sources and depots of a-syn in the blood [40]. Nevertheless, as already discussed above, it is still unclear if L1CAM-EVs exclusively originated in the CNS.
These findings were also described in the same publication in a patient-based study: PD patients showed significantly higher concentrations of total a-syn in EVs with putative neuronal origin compared to healthy controls. In contrast, the total a-syn amount in the plasma was similar among all individuals [32]. The sensitivity and specificity of plasma EVs a-syn were at a moderate level, but the total a-syn levels in those vesicles showed a weak yet significantly positive correlation with the Unified Parkinson’s Disease Rating Scale (UPDRS) motor scores and, therefore, with disease severity [32]. The exact entity of EVs entering from the CNS to the blood remains unclear, but different mechanisms than just secretion seem plausible. As observed in neurodegenerative disorders like PD [30, 31], damaged or dysfunctional BBB could further facilitate EV transfer. This trend of increased total a-syn in plasma L1CAM-precipitated EVs is in contrast to decreased levels of total a-syn in the CSF, which was also shown in different studies [45]. It seems possible that neuronal EVs detected in the blood may indicate the presence of some transfer mechanisms for transporting toxic, aggregated a-syn outside the CNS, which is responsible for the decrease of a-syn in the CSF. However, these mechanisms are still not fully understood, as a-syn in the CSF could also be delivered and retained in the brain, consequently showing decreased levels in the CSF [45].
In 2018, Zhao et al. also reported those trends of total a-syn in blood-EVs and detected another possible biomarker, the protein DJ-1 [42]. Oxidized DJ-1 protein, which is highly correlated with PD pathogenesis, was found in the brains of patients with idiopathic and early-onset familial forms of PD and was increased in neuron-derived EVs of the before-mentioned patients [46]. There is a positive correlation between DJ-1 and a-syn in neuron-derived EVs, although the relationship between those two biomarkers is unknown [42]. In another study, Si et al. [43] could even show different a-syn levels depending on disease onset and motor symptoms, which points to the possible versatility of this biomarker [43]. In line with these results, it was recently shown that L1CAM plasma-derived EVs from PD patients express significantly higher pathological forms of a-syn than the age-matched control patients. Moreover, by performing a seeding assay, it was also demonstrated that a-syn present in these EVs, but not in the whole plasma sample, can be amplified and seed pathological folding of a-syn [44].
Cerebrospinal Fluid
The extraction of CSF is not performed on a daily basis as a diagnostic procedure, for its associated risks accompanying the lumbar puncture, making it an interesting but not always available biomarker resource. a-Syn in CSF seems to be a valid disease marker, as oligomeric and phosphorylated forms of a-syn are increased in PD patients when compared to control individuals, while the level of total a-syn is decreased [47, 48]. EVs isolated from CSF of PD patients showed significantly lower levels of total a-syn and/or aggregated a-syn. Interestingly, this analysis was associated with better diagnostic sensitivity and specificity than the total analysis of a-syn in CSF [49].
Moreover, a panel of five miRNAs, in detail Let-7f-5p, miR-27a-3p, miR-125a-5p, miR-151a-3p, and miR-423-5p [50], have also been described in CSF-derived EVs as potential biomarkers, showing high sensitivity and specificity for PD diagnosis. In PD patients, EV-derived miRNAs related to the dopaminergic synaptic system and the neurotrophin signaling pathways were enriched [50]. Furthermore, the transcriptomic analysis of mRNA isolated from those EVs revealed a significantly reduced mRNA expression of several proteins, as shown for the amyloid precursor protein (APP), a-syn, and DJ-1 [51]. miRNAs were not only found in CSF-derived EVs, but altered levels of these transcripts were also described in blood-derived EVs [52]. In Cao et al., [52] specific miRNAs from EVs in serum could be detected and showed specific trends in PD patients [52]. Although these findings of miRNA alteration are intriguing and promising, further studies are needed with large cohorts to prove the sensitivity of those specific miRNA-markers and not sufficient knowledge of how the profiles differ from other synucleinopathies with similar motor manifestations.
Urine
Urine is easily accessible and therefore possesses a high potential for diagnosis and searching for possible biomarkers. Wang et al. showed increased phosphorylated disease-associated proteins in urinary EVs of PD patients, particularly the leucine-rich repeat kinase 2 (LRRK2) [53]. In PD, LRRK2 shows higher levels of autophosphorylation at serine 1292 (pS1292) induced by LRRK2-missense mutations, which are associated with neurodegeneration and late-onset PD due to neurotoxicity of pS1292-LRRK2 protein [53]. LRRK2 is expressed in collecting duct epithelial cells of the kidney, therefore, LRRK2 protein is present in high levels in EVs isolated from urine, however LRRK2 levels were highly variable in urinary EVs [54].
Although it would be very accessible in a clinical context, LRRK2 expression or other PD-related marker in urinary EVs are not yet sufficiently described for standardized use in PD diagnostic.
Saliva
Saliva appears to be advantageous because of its easy accessibility and absence of blood contamination.
Cao et al. [55] isolated EVs from PD patients' saliva and detected increased levels of oligomer forms of a-syn and an increased oligomer/monomer a-syn ratio in PD patients compared to healthy controls. At the same time, the total a-syn amount between the two study groups showed no significant difference [55].
While these promising results obtained from saliva samples could underline the importance and potential of this biofluid as a source of biomarkers in PD, collecting saliva from symptomatic PD patients is often challenging since hyposalivation is a common symptom of the disease. However, any artificial stimulation of secretion could change the composition of the secreted saliva, thus altering the results [48].
Conclusion
Although the exact mechanisms are still elusive, recent research shows that EVs play an essential role in neurodegenerative disorders. Besides potentially contributing to spreading of the disease, they are also promising tools for various diagnostic approaches. Neuronal EVs have a high potential to serve as biomarkers for PD. Their collection from the blood is advantageous and could make PD diagnosis more independent of clinical symptoms, varying daily conditions of patients, and the investigators' subjectivity. Several studies showed significant results for blood-derived EV-based diagnosis of a-syn and other biomarkers. Their findings could be integrated into a clinical context correlating to disease severity. Additionally, EV-purification from different biofluids has to be further investigated and standardized in the future since each biofluid has its assets and drawbacks. Blood seems to be the most accessible biofluid because its collection is routine in a clinical setting. As a source for a-syn analytics, blood-derived EVs show significantly better diagnostics and disease severity management results than non-EV-derived a-syn species from the blood. Also, EVs purified from CSF have emerged as a potential reliable biomarker, limited by the relatively complex and potentially harmful extraction of CSFs. While a collection of saliva might seem more accessible, it is often challenging due to hyposalivation, a common symptom related to PD. However, research focusing on exploiting the potential of saliva samples is highly warranted, since in EVs isolated from PD saliva samples, increased misfolded a-syn levels were detected. While urine has also been evaluated for EV-based diagnosis, identifying reliable and reproducible disease markers from this biofluid needs further studies. To sum it up, EVs in different biofluids, especially in blood, have the potential to be a reliable additional tool in PD diagnosis in the future but still need to be seen contextually with the clinical condition of the patient.
Moreover, it also has to be mentioned that techniques to purify and isolate CNS-derived EVs still need to be standardized and simplified, especially regarding neuronal markers, so that they can be used in a high-throughput clinical setting being applied in hospitals and laboratories.
Acknowledgments
This project has received funding from the Interdisciplinary Center for Clinical Research (IZKF) at the University Hospital of the FAU University of Erlangen-Nuremberg (Jochen-Kalden funding program N8) and the European Union's Horizon 2020 research and innovation program under the Marie Sklodowska-Curie grant agreement N°101030402.