Background
Extracellular vesicles (EVs) are a highly heterogenous group of cell-derived membrane structures 1. Based on their biogenesis, EVs can be classified into different subtypes including exosomes and microvesicles 2. To date, there is no specific surface marker to distinguish between the different EV subtypes. Furthermore, given cells may release differently assembled EV subtypes 3–6.
EVs contain a variety of bioactive molecules such as proteins, nucleic acids, and lipids. Their composition presumably depends on the cell of origin and its physiological condition 7. Therefore, EVs have attracted broad interest as intercellular communication vehicle, disease biomarker, and therapeutic agent 8–11. However, no standard EV protein analysis assay exists that is fast, sensitive, and yet feasible to be implemented in most laboratories for high-throughput analysis 12. Traditional methods like Western blot, typically require large sample volumes and significant processing. Flow cytometry is a powerful tool but its straightforward applicability for EV studies is hampered by the small size, low refractive index, and polydispersity of exosome-sized EVs 13–15. Thus, more advanced approaches like high resolution and imaging flow cytometry have emerged 16,17. Although these methods are extremely sensitive and allow the analysis of EVs at a single level, they tend to be laborious and require specialized setups as well as extensive operator expertise 18.
Here, we report a method to rapidly analyze EV proteins from human and mouse samples in a semi-quantitative way by conventional flow cytometry 19.
Methods and Materials
Cells and cell culture
Cell lines were grown at 37 °C, 5 % CO2 in a humidified atmosphere. HEK293T cells were cultured in DMEM 10 % FBS, 1× Antibiotic-Antimycotic (Anti-Anti). Immortalized, human bone marrow-derived mesenchymal stromal cells [hTert + mesenchymal stromal cell line (MSCs)] were grown in RPMI-1640 10 % FBS, 10−6 mol/L hydrocortisone and 1× Anti-Anti. IGROV1 cells were cultured in RPMI-1640 10 % FBS and 1× Anti-Anti. PANC-1 cells were cultivated in DMEM/F12 10 % FBS. To harvest conditioned media (CM), cells were grown in OptiMEM for 48 h.
Cerebral spinal fluid (CSF) samples
CSF samples were collected from patients at Karolinska University Hospital by lumbar puncture after informed consent and approval by the local ethics committee. Samples were pre-cleared by centrifugation (400 × g for 10 min, then 2,000 × g for 10 min) and filtered through 0.22 µm syringe filters with cellulose acetate membrane.
Human blood samples
Blood samples were drawn from donors after informed consent and approval by the local ethics committee. 6 mL samples of blood were isolated in heparin and serum-separating tubes. The blood was spun at 2,500 × g for 20 min twice with the platelet poor plasma being isolated. Samples were frozen at -80 °C or kept at 4 °C and run through size exclusion chromatography columns following manufacturer’s instructions.
Mouse experiments
All experiments were approved by the local board for animal welfare. For immunomagnetic enrichment of CD81 mouse EVs, citrate plasma (100 or 300 µL) was incubated with 50 µL CD81 Exosome Isolation MicroBeads for 1 h. Magnetically labeled EVs were applied to a µ Column placed in the magnetic field of a µMACS™ Separator and washed. Labeled EVs were eluted with 100 µL PBS after removal of the column from the magnetic field and further analyzed.
Female NMRI mice were intravenously injected with 2 × 1011 hTert + MSC-EVs in 100 µL PBS. Blood was sampled by heart puncture 1 min after injection and collected into PST-tubes following manufacturer’s instructions. Samples were depleted from cells by centrifugation at 2,000 × g for 10 min. After filtration, 120 µL plasma was transferred to the MACSPlex Exosome assay.
EV isolation and enumeration
For the analysis of cell culture-derived EVs, EVs were prepared from supernatants of HEK293T cells, MSCs, IGROV1, or PANC-1 respectively. Cells and larger debris were depleted by centrifugation (10 min at 500–900 × g, then 10–20 min at 2,000 × g). Subsequently, samples were filtered through 0.22 μm filters and EVs were concentrated by ultracentrifugation (2 × 110,000 × g). Sample input of EVs was determined by nanoparticle tracking analysis (NTA).
EV analysis with the multiplex bead-based platform
120 µL samples were loaded onto 96-well 0.22 µm filter plate. To each well 15 µL of MACSPlex Exosome Capture Beads were added and plates were incubated on orbital shaker overnight (14–16 h) at 450 rpm at room temperature. Subsequently, the beads were washed in PBS and resuspended in 135 µL of MPB. EVs bound by capture beads were stained for 1 h at room temperature with 15 µL of MACSPlex Exosome Detection Reagent cocktail compromising CD9/CD63/CD81-APC antibody conjugates. After staining, beads were washed and analyzed by flow cytometry.
Flow cytometry analysis
Flow cytometric analysis was performed with a MACSQuant Analyzer 10 flow cytometer. Samples were mixed before 70–100 µL were loaded to and acquired by the instrument, resulting in approximately 7,000–12,000 single bead events per well. Data were exported to comma separated files, which were subsequently imported into MATLAB for further analysis and visualization.
Results
A multiplex bead-based assay to analyze EVs using flow cytometry
The multiplex assay comprises 39 bead populations distinguishable by flow cytometry. Each of these capture bead populations is coupled to an antibody specifically targeting one of 37 exosomal surface epitopes or two isotype negative controls. After incubation with EV-containing sample, the EVs are stained with a detection reagent, e. g., a cocktail of APC-conjugated antibodies against the tetraspanins CD9, CD63, and CD81, which are commonly expressed on EVs. Consequently, sandwich complexes are formed between the i) capture bead, ii) EVs, and the iii) detection reagent. Then, a semi-quantitative analysis of EV surface markers is performed by flow cytometry resulting in a characteristic surface profile of an EV sample (fig. 1).
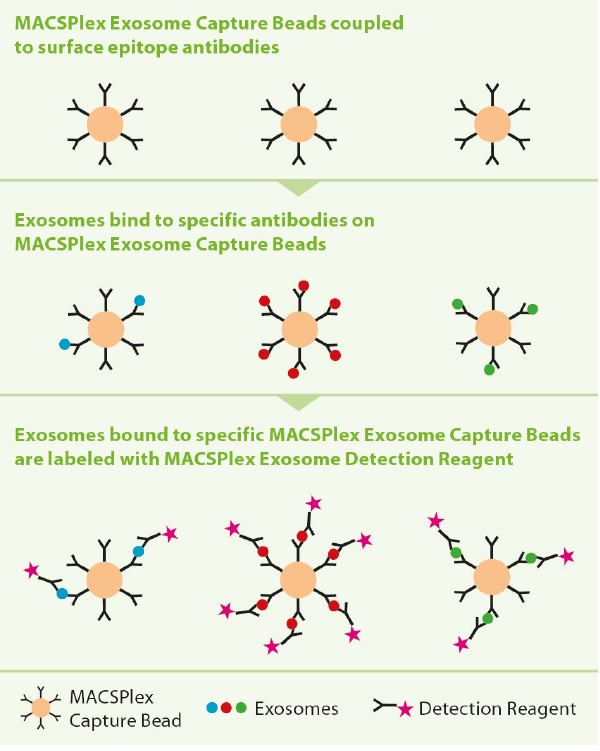
Figure 1: Principle of MACSPlex Exosome Kit. MACSPlex Capture Beads contain a cocktail of various fluorescently labeled bead populations, each coated with a specific antibody. Bound EVs on the beads are stained by a detection reagent and will generate a signal that is detectable by flow cytometry.
The bead-based assay represents a sensitive semi-quantitative method for EV analysis
The signal intensity detected with the detection antibodies depends on the EV concentration. To assess the range of detection, we defined 5 different doses of small EVs based on particle concentrations measured by NTA (1 × 108 to 1 × 106) isolated from HEK293T cells. As expected, signal intensities for positively stained bead populations decreased with decreasing EV input. The tetraspanin markers CD9, CD63, and CD81 could be detected with only 1 × 106 particles. Less abundant markers, like CD29 and CD49e required higher EV inputs for reliable detection. At high doses (1 × 108 particles), some markers were detected, e. g. CD24 and MCSP, which were close to background levels at lower doses (fig. 2).
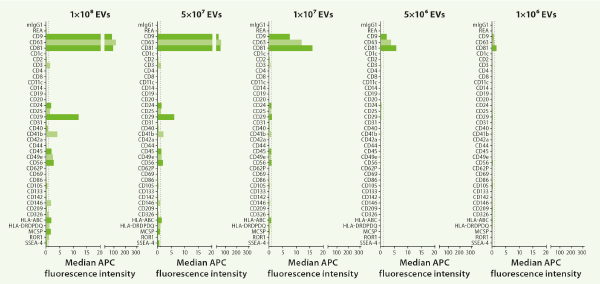
Figure 2: Detection range of the MACSPlex Exosome Kit. EVs were prepared from HEK293T CM. Subsequently, the performance of the assay was evaluated for input ranges between 1 × 106 and 1 × 10⁸ particles, as determined by NTA. EV surface marker compositions after CD9/CD63/CD81-APC detection are shown. Data were adapted 19.
Together, the bead-based EV assay enables a sensitive semi-quantification of EV surface markers.
Custom detection antibodies are compatible to analyze EV surface epitope expression
The assay enables the analysis of 37 EV surface epitopes but whether additional surface markers are present on EVs will likely be a recurring question. Therefore, we tested an antibody against folate receptor 1 (FOLR1) as staining reagent. FOLR1 on EVs was reported to shuttle folate into the brain and is examined as a biomarker for ovarian cancer 20,21. EVs from pre-cleared cell culture supernatant of the human ovarian adenocarcinoma cell line IGROV1 showed robust expression of abundant markers like tetraspanins, the integrins CD29 and CD49e, and other epithelial markers like CD326/EpCAM (fig. 3), when the detection reagent cocktail was used.
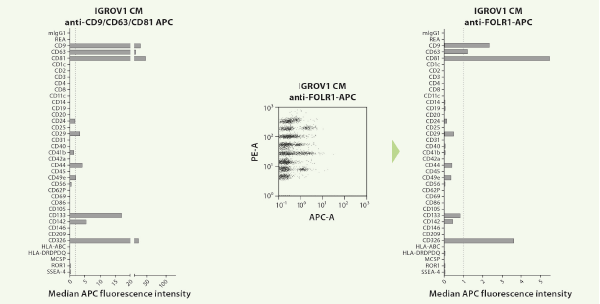
Figure 3: Compatibility of the MACSPlex Exosome Kit with anti-FOLR1-APC as detection antibody. EVs in CM from cultured IGROV1 cells were analyzed using the MACSPlex Exosome Kit and stained with the CD9/CD63/CD81-APC detection cocktail contained in the kit (left) or alternatively with the APC-conjugated anti-FOLR1 antibodies (right). The epitopes on the bar chart indicate the specificities of the MACSPlex Exosome Capture Beads. Bars show the median fluorescence intensity of APC. Data were adapted 19.
When using anti-FOLR1-APC as detection antibody, signals for the CD9, CD63, CD81, and EpCAM capture bead populations were gained (fig. 3). This indicates that the EVs express FOLR1 and that those EVs co-express the tetraspanins and CD326. Our data also highlights that custom detection antibodies are compatible with the assay.
The EV surface profile of different biological fluids can be assessed
We tested the assay to detect EVs in human CSF, plasma, and serum. Results were visualized as heatmaps for comparison (fig. 4).
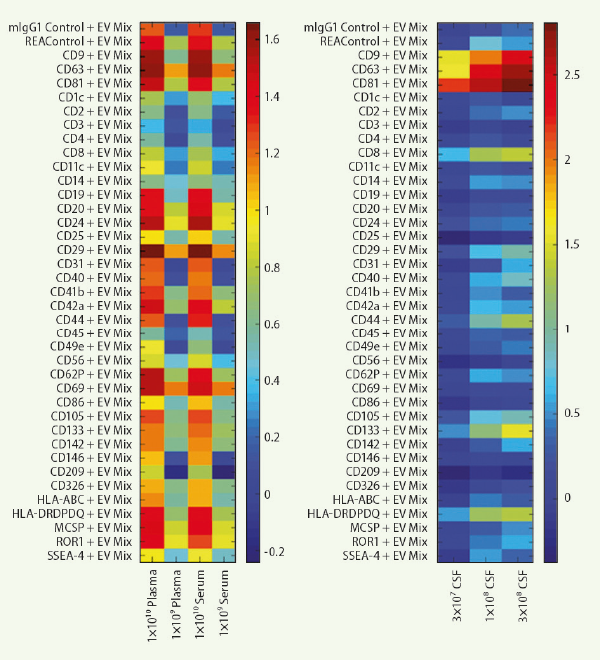
Figure 4: Analysis of human biological fluids with the MACSPlex Exosome Kit. Pre-cleared CSF, non-purified plasma, and serum samples with different input amounts were analyzed. Heatmaps of surface marker detection on CD9+/CD63+/CD81+ EVs are shown. Data were adapted 19.
High and consistent levels of tetraspanins were detected in pre-cleared CSF samples, in non-purified plasma, as well as in serum, with no major difference among EV surface marker profiles in the latter two sample types (fig. 4). Consistent with previous reports, the stem/progenitor cell marker CD133 and HLA-DRDPDQ were highly expressed on CSF EVs (fig. 4).
In summary, this multiplex bead-based assay is useful to characterize the general EV surface marker composition in different body fluids.
Native, non-manipulated human EVs can be detected in animal models
The therapeutic application of EVs has emerged to combat cancer and other diseases. Several animal studies reported the treatment with EVs as being effective and safe. However, the precise mechanism of action of EVs remains poorly understood, partially due to the technical limitations in tracking administrated EVs over time. We hypothesized that the human specific multiplex assay could be useful to analyze human EVs after injection into mouse models. Briefly, we isolated EVs from an immortalized human MSC line, injected doses of 2 × 1011 EVs/mouse intravenously and took blood samples after 1 min. Undiluted plasma samples were analyzed in the multiplex flow-cytometry assay. While no signal was observed in plasma from non-injected control mice, clear signals for distinct EV surface markers could be detected for injected mice (fig. 5).
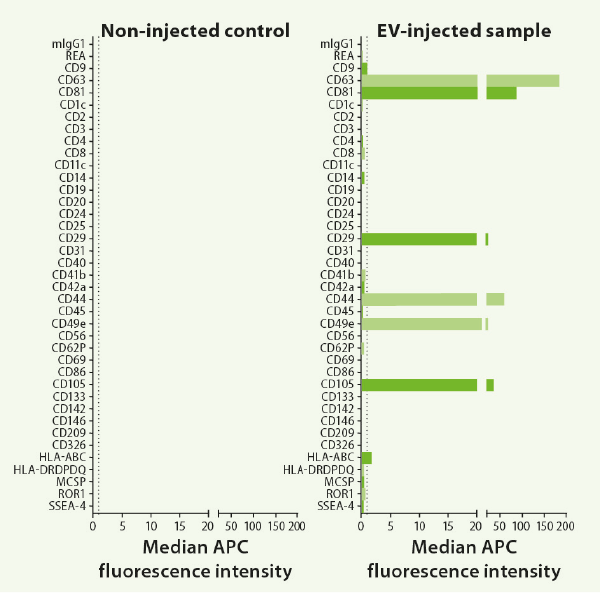
Figure 5: Detection of human EVs in mouse blood with the MACSPlex Exosome Kit. Mice were intravenously injected with 2 x 1011 particles. Blood was taken 1 min after injection and plasma was analyzed. EV surface marker composition after CD9/CD63/CD81-APC detection is shown for non-injected (left) versus injected (right) blood sample. Data were adapted 19.
Analysis of EVs isolated from plasma with superparamagnetic MicroBeads using the MACPlex Exosome Kit, mouse
EVs were enriched from 300 µL or 100 µL of mouse plasma using CD81 Exosome Isolation MicroBeads and compared to EVs from 300 µL plasma without pre-enrichment. We detected small amounts of CD63- and CD81-positive EVs indicating exosomal origin of the captured vesicles. Of note, the CD81 epitope on EVs is blocked after EV isolation with CD81 MicroBeads and therefore cannot be detected. Common markers like CD31, CD40, CD44, or CD49f indicated that some EVs were of endothelial or epithelial origin. Other markers implied that EVs originated from leukocytes (CD45) or platelets (CD41, CD62P). The marker profile was similar for EVs from unseparated plasma and pre-enriched CD81-positive EVs. Sensitivity increased by about 3-fold after pre-enrichment, thus enabling a multiplex analysis from as little as 100 µL of mouse plasma (fig. 6).
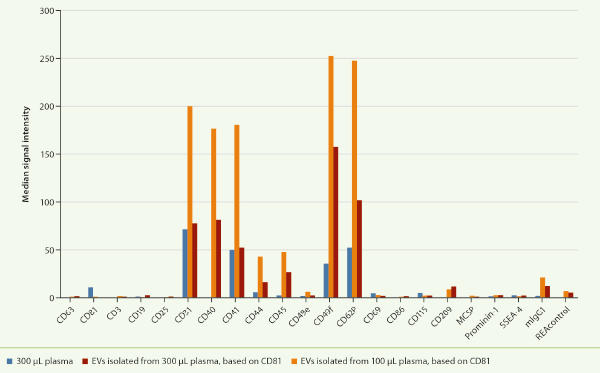
Figure 6: Analysis of EVs isolated from plasma using supermagnetic MicroBeads. EVs enriched from mouse plasma (300 μL or 100 μL) using CD81 Exosome Isolation Microbeads were compared to EVs from plasma without pre-enrichment. EV surface marker composition after CD9/CD63/CD81-APC detection is shown.
Discussion
The multiplex-bead-based flow cytometric assay facilitates specific and robust semi-quantification of EV surface antigen profiles in different types of samples. These include unprocessed cell culture supernatants or cell culture-derived EVs from cancer cells 22, fibroblasts 23,24, endothelial 25 and stem cells 24,26,27, as well as body fluids, such as CSF 28, plasma 29,30, urinary and saliva 31. The assay works on any flow cytometer with a blue and red laser and does not demand extensive operator expertise. Thus, it can be easily implemented in most laboratories for quality control and routine analysis of EV samples. The bead-based platform is further suitable to detect heterogeneity within one EV sample and in combination with other technologies supports EV analysis at single vesicle level 5. Under standardized condition it represents a valuable tool to compare EVs from different sources. This allows to characterize the general repertoire of surface markers expressed on clinically relevant EV samples. Thereby, the assay offers the promise to unravel EV markers specific for certain cell types and diseases.
The assay can be adapted to individual needs. Instead of the tetraspanin antibodies, custom detection antibodies can be used to test the expression of additional EV surface markers
The therapeutic role of EVs has been intensively investigated in animal models of various diseases, such as cancer or degenerative diseases, respectively. The possibility to specifically identify human EVs in the blood of EV-injected mice would add an additional level of depth to current biodistribution and pharmacokinetic studies, which rely on labeled EVs and often fail to assess surface signatures. It further opens up new avenues to study non-manipulated EVs in xenograft tumor, organ or cell transplantation models.
Overall, the multiplex exosome assay introduced here could be a tool to facilitate standardized EV analysis and support insight into their biological functions.
André Görgensa,b *, Oscar P. B. Wiklandera,
R. Beklem Bostancioglua, Antje M. Zicklera,c, Florian Murkeb,
Ute Heiderd, Stefan Wildd, Bernd Giebelb and Samir EL Andaloussia
a Clinical Research Center, Department of Laboratory Medicine,
Karolinska Institutet, Stockholm, Sweden
b Institute for Transfusion Medicine, University Hospital Essen, University of Duisburg-Essen, Essen, Germany
c Division of Pathology F56, Department of Laboratory Medicine,
Karolinska Institutet, Karolinska University Hospital Huddinge, Stockholm, Sweden
d Miltenyi Biotec BV & CO. KG,
Friedrich-Ebert-Str. 68, 51429 Bergisch Gladbach, Germany
* Corresponding author