Digitization in biobanking: Where to find it and what can we expect?
DOI: https://doi.org/10.47184/tp.2024.01.02In recent years, the introduction of a broad spectrum of digital technologies has changed various workflows and the day-to-day business of biobanks. There are many systems and technologies that can be used in biobanking procedures, medical research, and practice. These digital technologies can bring significant benefits, but also great responsibilities. This report briefly discusses the digitization of biobanking processes and outlines some challenges and possible future perspectives.
Keywords: Biobank, digitization, digital biobanking, biomaterial, biobank workflow, sample collection
Where can we find digitization in biobanking workflows?
Digitization can impact the entire life cycle of the biomaterials, from collection to its storage and retrieval for biomedical research (Fig. 1).
Many systems and technologies have fundamental effects with potential benefits for biobanking procedures, medical research, and practice. However, some of them carry great responsibility [1]. So, where do we find these systems, technologies, and digitization in biobanking workflows? Even prior to collection digitization can streamline the workflow. Patient's consent to the collection, storage and utilization of their biomaterial and data for research purposes is often still obtained in paper form. The use of an electronic broad or informed consent simplifies the process, and the specific points to which the patient has consented could be stored directly in the clinical information management system [2]. Future consent management may even offer patients the option of dynamic consent control. In this field , efforts are underway to provide consent management platforms that allow patients to change their personal and contact details , adjust their consent decisions and track the progress of research projects that they have supported with biomaterial and/or data [3, 4].
The automation options for the entire biobank workflow have been widely accepted as necessary for standardizing sample collection processes and thereby optimizing sample quality. Options range from automated transport by actively moving sample carriers, automated liquid handling by sample aliquotation systems, up to automated freezing/storage systems with several systems on the market [5]. This automation provides the possibility to digitally track the entire sample processing, collecting important data on pre-analytical handling and storage conditions. Registration of the sample collection tube can be done directly at the collection site using barcodes or QR codes and can be linked to pseudonyms on e. g. patient wristbands. This links the sample directly to the patient and reduces the risk of sample mix-up. Transport from the collection site to the biobank can be monitored by temperature logging tools, and automated sample handling can track processing, final freezing, and storage time points [6]. Radio Frequency Identification (RFID) tags have been described as an alternative to barcodes or QR codes. They can contain more information and do not require a direct line of sight to read. They can be placed inside the transport box, storage tube or cryotube next to the sample, even inside liquid samples or tissue cassettes, collecting data on transport and storage temperature on the way to the biobank or during sample processing [7].
Temperature fluctuations during both the pre-analytical phase and storage time are important information as they can reduce sample quality by affecting various metabolites [8, 9]. In terms of sample storage, a recent study revealed different temperature zones and temperature deviations in ultra-low temperature freezers (ULTFs; ≤ -80 °C) [10]. Digital real-time monitoring provides a practical tool for temperature control [11] and is often already integrated into modern ULTFs and nitrogen-based storage systems. In addition, the closed semi-automated or fully automated nitrogen-based storage systems identify, store, and retrieve samples on a single-tube basis using pre-registered tubes with barcodes or QR codes for direct sample tracking. Future setups may include augmented reality glasses (AR-glasses) to facilitate sample picking or rearrangement in ULTFs using digital lists [12].
Tissue collecting biobanks can also benefit from digital technologies in pathology. Formalin-fixed paraffin-embedded (FFPE) tissue sections can be fully digitized as whole slide images (WSI) using small or high throughput scanner systems. Images can then be stored in an image database with annotations and pathology information. These digital images can be used directly for research purposes and analyzed using AI-based models, deep learning or machine learning models for object identification, segmentation or classification [13–15].
Depending on their integration into the clinical setup, modern biobanks are able to digitally collect and combine different types of information, i.e. patient data, consent data, clinical data, biomaterial data and analytical data, shifting from the classical view of a biomaterial repository to a bridge between biological and digital data [14]. Biobank Information Management Systems (BIMS), specifically adapted to biobank workflows, are regarded as one of the necessary technological requirements of a modern biobank. Registration of a sample and storage of corresponding data are core functions that allow the integration of multiple information throughout the processing workflow. Each step is recorded by a custody log ensuring transparency and traceability. Many BIMS additionally offer the capacity to store analytical data and integrate and organize multiple datasets into a single, searchable framework [16]. The real potency of these diverse datasets can be achieved by combining all the information from multiple sources, including patient lifestyle, disease information and “omics” data, with the biomaterial and aggregating this data [1, 16]. IT networks and setup of web-based portals such as the “Sample Locator” or the combination of data integration centres and biobanks into a data exchange platform [17] are powerful options to generate a link between biobanks and researchers, facilitating the search for biomaterial and data to support high-quality research. In the future, the nationwide and even international connection of different IT infrastructures will further push research about e. g., new disease treatment strategies or drugs, to the next level [18].
Finally, it is important to provide future healthcare professionals with basic knowledge of biobanking, as they will be obtaining patient consent and, in some cases, dealing with pre-analytical factors, biomaterial collection and data. Here, the use of digital learning platforms can support and complement the implementation of educational programmes [19].
Difficulties and Challenges
Digitization is often associated with significant costs. This includes the purchase of new equipment, software or upgrades. The use of RFID equipment is more extensive compared to classical barcodes and the error rate (unreadable samples) needs to be reviewed [7]. Manual data insertion is time consuming and error prone and should be avoided. Data heterogeneity and quality issues need to be addressed, which is challenging, as data formats are often different, missing its full potential while channeled through algorithms. An international standard data format is needed, as well as a reliable infrastructure with fast and secure interfaces for management and maintenance, focusing on interoperability between different hardware and software systems. Most importantly, we must ensure compliance with the principle of equity, privacy, secure biomaterial and data handling, and secure storage to trace a sample back to the patient. Biobanks need to prevent misuse of biomaterials and data, and the generation of multiple digital copies for data backup can complicate complete deletion after a patient's content has been withdrawn [1, 6, 16].
With respect to the present difficulties and challenges, a well-trained, multidisciplinary team is required to ensure a successful transformation towards digitization in biobanking (Fig. 2).
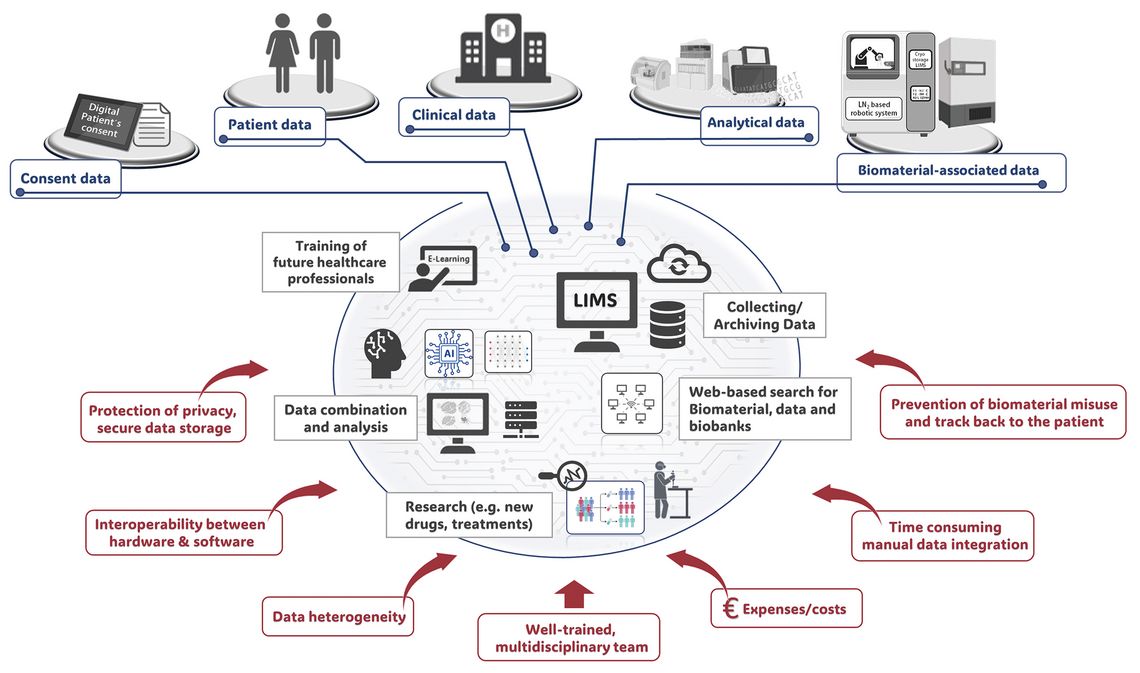
Figure 2: Digital biobanking – transformation from classical biomaterial repositories into powerful research support sites by collecting, archiving and combining different data, e.g. patient data, consent data, clinical data, biomaterial data and analytical data. AI-based aggregation of the various data types can successfully support research. Figure was created using Microsoft Power Point and the BioRender.com software.