Extracellular vesicle-derived microRNA biomarkers: goals and pitfalls
Liquid biopsy-derived extracellular vesicles (EVs) are an auspicious source for transcriptomic biomarker studies. Here, we review the potential of EV microRNAs (miRNAs) biomarkers, exemplary outline commonly used methods to elucidate new biomarker signatures, and pivotally discuss their applicability at present.
Keywords: extracellular vesicles, liquid biopsies, transcriptomic biomarkers, microRNAs
Molecular biomarkers
A biomarker is a “defined characteristic that is measured as an indicator of normal biological processes, pathogenic processes, or responses to an exposure or intervention, including therapeutic interventions” [1]. Biomarkers should be directly associated to a certain disease state or condition, allowing for precise, reproducible and fast evaluation of molecular alterations in an objective manner [2, 3]. Multiple marker signatures are preferred over single biomarkers [4], as analytical specificity strengthens the diagnostic, prognostic or monitoring potential.
The development towards the routine use of high-throughput molecular techniques, like next generation sequencing (NGS) or proteome profiling, led to a massive increase in potentially valuable molecular biomarkers [2]. But up to now, their clinical implementation is still limited. More than 150,000 biomarker studies have been published by 2011 from which only around 100 reliable biomarkers have been successfully transferred for clinical routine use to the hospitals [5].
In this review, we want to address the goals and pitfalls of extracellular vesicle (EV)-derived microRNA (miRNA) biomarkers in the context of molecular diagnostics.
Transcriptomic biomarkers
Why do we focus on RNA – also referred to as transcriptomic – biomarkers? As compared to DNA, besides genetic insights, the transcriptome provides dynamic information on cellular states and regulatory processes [6]. First early response genes, like transcription factors, are activated within 10 to 20 minutes, after hours mid to late response genes are transcribed. Thus, quantifying RNA biomarkers enables an earlier evaluation of cellular physiological changes, disease states or conditions than measuring proteins.
Moreover, transcriptomic biomarkers show higher sensitivity and specificity, and are more cost-efficient than proteomic markers. Nucleic acid biomarkers are easy to quantify at very low cellular abundance levels by either polymerase chain reaction (PCR) [6, 7], such as reverse transcription real-time PCR (RT-qPCR), or RNA sequencing (RNA-Seq). RNA-Seq analyses can be performed comprehensively, since all transcripts are measurable in parallel in one run [8], thereby reducing possible batch effects. Additionally, good pathway and network interaction tools are available for the RNA world, e. g. DIANA tools [9] (www.microrna.gr).
But what are the advantages of miRNAs over other transcriptomic biomarkers? Short is better – miRNAs have exceptional high stability [10], unlike longer RNA classes, as for example messenger RNAs (mRNAs) or long non-coding RNAs (lncRNAs). The potential of other small regulatory RNA classes, such as piwi-interacting RNAs (piRNAs) have not yet been thoroughly investigated and only few biomarker relevant articles have been published so far [11]. Although miRNAs and lncRNAs have both been discovered almost 30 years ago [7, 12], miRNAs have been studied more extensively as potential biomarkers than lncRNAs [13], and therefore more extensive miRNA databases are currently available, e. g. miRBase [14] (www.miRbase.org) and miRDB [15] (www.miRDB.org). Additionally, miRNAs are protected from endogenous RNase activity by being encapsulated in EVs or by forming complexes with different kinds of RNA-binding proteins, such as Argonaute proteins or high-density lipoproteins [7, 16].
Liquid biopsies
The availability of high-throughput and highly sensitive methods enables the detection of nucleic acid biomarkers even in low concentrated or low volume patient samples. This allows for minimally invasive sampling of body fluids like blood, urine and saliva amongst others [17]. Liquid biopsies, as these sampling methods are called, offer the possibility to detect diseases in very early stages or in patients where conventional tissue biopsies cannot be performed [17–19]. Furthermore, liquid biopsies make it possible to take multiple samples at any point of time in one patient, to monitor the stage or progress of the disease or follow the therapy success [17, 18]. Because these are crucial advantages for the successful treatment of numerous diseases, e. g. in most types of cancer, liquid biopsies have become quite popular in recent years.
In liquid biopsy samples different targets can be used for molecular diagnostics (Table 1), e. g. circulating cell-free DNA (ccfDNA), circulating RNA, circulating tumor cells (CTCs), and EVs [17].
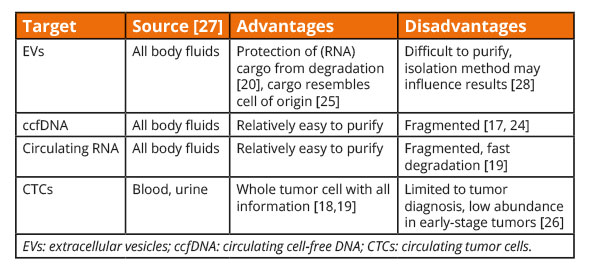
EVs and more specifically their miRNA content, being present in all kinds of body fluids, are an excellent and often chosen target for liquid biopsy analysis [17, 20]. While circulating nucleic acids are relatively easy to purify from blood samples, EVs are not. EV isolation methods often co-isolate contaminants, most notably various lipoproteins, which are similar to EVs in size and density [21] and may interfere with downstream analysis. Although the choice of purification method can influence the results of EV analysis, a consensus on EV isolation has not yet been established [22, 23].
However, circulating free RNA or DNA is rapidly degraded by nucleases in the extracellular space [19]. Although ccfDNA is more stable than RNA, due to its protection by nucleosomes or other proteins, it is also subject to degradation and fragmentation [17, 24]. Inside EVs, however, nucleic acids are protected from nucleases by the vesicle membrane [20]. Furthermore, the EV cargo is similar in composition to the original cell, meaning that EVs shed by cells involved in a certain disease contain a biomarker signature closely related to the disease [25].
CTCs, on the other hand, are cells that disassociated from the original tumor and therefore carry its mutations and biomarkers [18, 19]. However, they are primarily found in blood and urine and only focus on tumor diagnosis [17]. Furthermore, few CTCs are found in early stage tumor patients, limiting their potential for early diagnosis [26].
EV miRNAs as new avenues for transcriptomic biomarker studies
EVs participate in cell-cell communication amongst others by transferring miRNAs [25]. The discovery of functional mRNA and miRNA in EVs [29] opened up new avenues for transcriptomic biomarker studies that offer definite advantages. EVs are isolated from non-invasive liquid biopsies (I), are relatively stable (II), protect miRNAs from degradation (III), and are specifically loaded with miRNAs (IV) [30, 31]. EV-based RNA biomarkers would frequently be undetectable in unfractionated or unprocessed biofluids due to high, unspecific background signals. However, one must be aware that different purification methods may result in distinct EV subpopulations and may therefore effect the results of downstream RNA profiling [22, 23].
Liquid biopsies from blood appear to be a robust source for EV RNA research, as storage of plasma samples for up to 12 years at -80 °C, two weeks at 4 °C, two days at room temperature, and multiple cycles of thawing and refreezing, all resulted in intact, non-degraded EV RNA [32, 33].
Long-term storage and freeze-thaw cycles of EVs themselves seem to only marginally impact their amounts [34]. Additionally, EV-derived nucleic acids imply to be stable under various conditions, as for example storage of EVs for one week at 4 °C, one day at room temperature and repeated freeze-thawing, only minorly impact the stability of EV DNA [35].
Hence using EV-related miRNA for molecular diagnostics has major advantages over other target molecules [36]. A PubMed (www.pubmed.ncbi.nlm.nih.gov) search for “extracellular vesicle, microRNA biomarker” identified more than 1,200 publications (May 2020) related to various disorders including diverse types of cancers, neurodegenerative diseases and sepsis, indicative for the innovative exploration of EV miRNAs as biomarker source.
By now, a vast number of EV-related miRNAs has been associated with numerous diseases, one example being cancer. Many potential EV-associated miRNA biomarkers have been discovered for various types of tumors . To name just a few examples, the exosomal miRNAs miR-486-5p, miR-181a-5p and miR-30d-5p have recently been identified as circulating markers of high-risk rectal cancer [37]. Another example is miR-21, which has been shown to be involved in many types of cancer [38], e. g. glioblastoma [39], non-small-cell lung cancer [40] or hepatocellular carcinoma [41].
How to elucidate potential EV miRNA biomarkers
Molecular profiling of EV miRNAs from non-invasive liquid biopsies by high-throughput technologies, in particular small RNA-Seq, is a powerful platform to comprehensively screen for new disease-related miRNA biomarker candidates (Figure 1) [8].
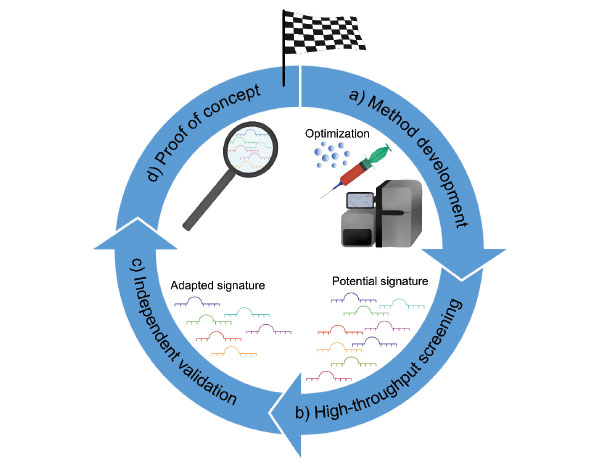
Although EVs are enriched for miRNAs in various physiological or pathophysiological conditions [20] and library preparations for small RNA-Seq typically include a size selection step to exclude larger RNA fragments (>200 nucleotides). NGS data from EV isolations can still include a considerable amount of non-miRNA sequences that need to be removed to reduce background noise and strengthen miRNA-specific disease- or treatment-related signals. Origins of these unspecific RNA fragments can be manifold and range from library preparation artefacts like sequencing adapter dimers to degradation products caused by handling or storage [8]. Furthermore, significant variance is also added to EV biomarker studies by vesicle isolation methods and the resulting prevalent subpopulations of EVs [42]. Researchers need to pay special attention during experimental setup to consider possible consequences on required sample sizes or sequencing depths arising from less pure but highly concentrated EV preparations such as precipitation methods compared to for instance size-exclusion chromatography [22].
Having navigated these pitfalls, researchers are rewarded with an extensive miRNA profile from EVs that often display much higher discriminatory power than comparable data sets from unfractionated and unprocessed liquid biopsies. By using different bioinformatical approaches of multivariate data analyses, or combinations thereof, a candidate biomarker signature is developed from miRNA expression data in a training set of individuals. Outliers or dominant batch effects can be visualized by unsupervised clustering methods such as hierarchical clustering analysis (HCA) and especially principal component analysis (PCA) or t-distributed stochastic neighbor embedding [43]. Differential gene expression (DGE) analysis detects the most drastically dysregulated transcripts between treatment groups [44], while sparse partial least squares discriminant analysis (sPLS-DA) reveals a subset of EV miRNAs efficient to discriminate all the available groups [45]. Resulting potential biomarker patterns can be cross-referenced with results from pre-existing studies collated in databases such as Vesiclepedia [46] or EVmiRNA [47] to validate specificity for tissues or diseases not included in the experimental setup. By expanding potential miRNA biomarker sets with their corresponding mRNA targets, functional and biological relevance can be assessed through gene enrichment analysis of disease-associated pathways. Databases for mRNA targets include computational prediction models [48] as well as repositories of experimentally validated mRNAs [49]. If available, integrated analyses of miRNA with mRNA or protein information can also be incorporated by directly obtaining data sets of comparable studies from publicly available data repositories like the European Nucleotide Archive [50] or the Sequence Read Archive [51].
Subsequently, the candidate biomarker signature is quantified in a validation trial, to proof for stability and reproducibility, and hence improve the diagnostic, prognostic or monitoring value of the EV miRNA profiles. Ideally, the EV miRNA signature is quantifiable in the same training set by using an orthogonal miRNA analysis platform, particularly RT-qPCR, and additionally confirmable in a larger, biologically independent validation set of individuals. Outsourcing of validation experiments to external laboratories allows for independent proof of validity of the EV miRNA biomarker signature [52].
A long and stony road to the applicability of EV miRNA biomarkers
Although we assume the transfer of EV miRNAs as messages from the “releasing cells” to the “target cells” in intercellular communication, there is a considerable knowledge gap. Cells secrete different subtypes of EVs with varying sizes and compositions, some of which are believed to not even contain RNA as a cargo [53]. The mechanisms which actually regulate miRNA loading into EVs and how these information influence recipient cells are still poorly understood. Whether these miRNAs are exclusively intraluminal, or maybe located at the outer EV membrane, is yet uncertain [54].
Affected by an irreproducibility problem [52], circulating miRNA biomarkers are currently rather non-specifically related to a multitude of disorders and outcomes, with few accordance between equal studies of even the same disease [55]. But what are the obstacles in the path generating this enormous diversity?
There is mostly an insufficiency of control for pre-analytical variables including collection, handling and storage of human biofluids, affecting both their quality and consistency, and in the same way the recovery of EVs, as well as the grade of potential EV miRNA biomarkers. Thus it is essential to process all samples identically and report all known pre-analytical parameters [52, 56].
The comparability between labs is often limited, as different miRNA analysis platforms with divergent sensitivities, settings and overall performance are available, thereby highly impacting results [57]. Additionally, diverse biofluids and starting volumes are the basis for a multitude of EV separation and RNA extraction protocols resulting in divergent EV miRNA expression profiles [22, 23].
To facilitate and improve standardization in the EV field, the MISEV guidelines were published and provide recommendations with regard to the minimal information for studies of EVs [56]. Other position statements from the International Society of Extracellular Vesicles (ISEV) focus on EV subtopics, for instance EV-associated RNA [54]. EV-TRACK offers a platform for transparent reporting and centralizing knowledge in EV research [28].
Now the question comes up: what is the best strategy for normalization? While some researchers apply a definite starting volume of the respective biofluid and thereof extracted EV total RNA, others consider EV numbers or RNA concentrations for their assays [58, 59]. However, as EVs are nanosized and carry only limited quantities of RNA, accurate quantification of EVs themselves and their RNA cargo by highly specific and sensitive techniques still remains challenging [54, 56]. Moreover, different amounts of miRNAs in EV subtypes might hamper normalization based on the vesicle level.
Currently, most EV miRNA biomarker studies are conducted in fairly small study populations, while generating truly homogenous cohorts can be challenging. It is well-known that miRNA expression is affected by biological properties such as sex, age or body mass index [60], and it is obvious that EV composition is influenced by demographics as well [61, 62]. As each patient represents an individual medical condition, prescribed treatments and medications may lead to distinct outcomes due to heterogeneous patient responses to therapy. Profiling of EV miRNAs is therefore recommended in large cohorts, to minimize the confounding effects of differential expression due to individual variability in both patients and healthy controls.
Conclusion
Despite the tremendous effort in EV miRNA biomarker research, there are currently no specific and validated targets with real potential for clinic routine settings available. High inter-lab variability is introduced by divergent pre-processing approaches of multiple human biofluids, various combinations of EV separation and RNA extraction methods, added by different miRNA analysis platforms, normalization, and data evaluation strategies.
A future focus on standardization of the EV-based transcriptomic biomarker workflow is urgently required. Increased efforts aiming to solve the challenges related to EV research are essential, filling the knowledge gaps by strengthening research, to exploit the opportunity of a new innovative approach of clinically applicable and non-invasive molecular biomarkers. Since single miRNAs are involved in many different pathogenic pathways, diagnostic strategies should focus on patterns of up- and downregulated miRNAs that are specific for certain diseases.