“Brainstorming”:
Extracellular Vesicles in Physical Activity and Neuronal Health
Physical and mental activity are known to contribute to brain health and overall longevity. Extracellular vesicles (EVs) have attracted attention for their ability to transport bioactive cargo through various body-fluids and their role in tissue crosstalk and regeneration. Targeted intercellular communication processes, including those facilitated by EVs, are of vital importance for the complex architecture and function of the brain. Activated neurons trigger the transfer of EVs from myelinating oligodendrocytes to neurons, promoting neuronal long-term maintenance and survival...
Keywords: oligodendrocytes, neuron-glia interaction, extracellular vesicles, physical exercise, neuronal maintenance, neuroprotection
Cellular architecture of the brain and glial support
The human brain is a complex organ, which consists of billions of cells with comparable amounts of neurons and glia cells. While interconnected neurons form broad signaling networks, glial cells control the function of these networks and contribute to the physiological balance (homeostasis) of the brain. Neurons are connected to each other via their synapses to form circuits that conduct and process electrical impulses at high speed to distant areas in the nervous system and peripheral tissues. The diverse abilities of glial cells are of fundamental importance for the everyday functions of the brain. They guide the development of neuronal circuits, accelerate and optimise signal conduction, regulate signal processing and control brain metabolism [1, 2]. Hence, continuous intercellular communication between neurons and glia is mandatory for correct neural development and function [3]. Reduced neural performance becomes visible when transmission of information between the cells is disturbed or inefficient, manifested e. g. in neurodegenerative diseases such as Alzheimer’s disease or multiple sclerosis. Unraveling the molecular mechanisms responsible for effective intercellular communication is, therefore, essential to understand the physiological and pathological processes in the brain as well as its maintenance during lifespan.
The special ability of neurons to conduct electrical signals rapidly over long distances is enabled by their long extensions called axons. However, impulse propagation is a highly energy demanding process associated with cellular stress. In particular, the areas along the axon projecting far away from the nourishing cell body depend on external support for their maintenance. Oligodendrocytes enwrap axons with an insulating myelin sheath, facilitating rapid impulse propagation. Moreover, oligodendrocytes play an important role after myelination has been completed. Various observations show that they ensure long-term integrity of axons at distant areas by providing trophic support [4]. Intriguingly, a defect originally caused in oligodendrocytes, such as PLP- and CNP-deficiency, leads to secondary degeneration of the axon established by increasing axonal swellings over time [5, 6]. This indicates that the cellular transport processes mandatory for axonal maintenance are disturbed leading to a ‘traffic jam’. Conclusively, local support of axons by factors provided by oligodendrocytes is required to keep axons healthy and functional. A detailed understanding of the underlying molecular mechanisms of this glial support is important to understand the requirements of long-term axonal maintenance. If these could be decoded more precisely, new targets for the treatment of neurodegenerative diseases or for counteracting the age-related decline of neurons could possibly be available.
Extracellular vesicles as complex messengers between cells
Cells communicate via the exchange of signaling molecules, which are recognized by recipient cells and translated into cellular responses. A non-canonical pathway of cell-cell communication is the transfer of compound biomolecular cargo by extracellular vesicles (EVs). Different types of EVs are formed by numerous cell types and a mixture of highly diverse vesicles can be found in various body fluids [7, 8]. Microvesicles directly shed from the plasma membrane, whereas exosomes are formed inside the cell within multivesicular bodies (MVBs) and then actively released into the extracellular space. These vesicles transport bioactive molecules (lipids, metabolites, proteins, as well as nucleic acids) and surface molecules that are specifically recognized by certain cells enable a targeted transfer of information [9, 10]. Overall, the structure and function of EVs is reminiscent of viruses. Instead of reprogramming their target cells in order to replicate, which often causes damage to the host cells, EVs do not replicate but make their contents available to the cell. Once taken up by the recipient cell, encapsulated cargo may sustainably act on the cellular fate by activation of signal transduction pathways or by modulating the protein composition either directly or via the transfer of coding and non-coding RNAs affecting protein synthesis [11]. Although the horizontal transfer of RNA species via EVs is widely studied, many basic questions are still open. Recent developments in EV characterization suggest that a careful re-evaluation of the reported RNA-mediated effects on cells may be required, since impurities may produce artefacts [12–14]. Furthermore, the molecular mechanisms of RNA sorting to EVs and the functional retrieval of EV-RNA in target cells, which is expected to be controlled by RNA-binding proteins, is not well understood. Interestingly, a recent study showed that the neuronaI retrovirus/retrotransposon-like protein Arc recruits into EVs RNA, which is transferred across synapses and undergoes activity-dependent translation, implicated in synaptic plasticity [15, 16].
Overall, the molecular composition of EVs is known to be dynamic and to reflect the cellular state. Hence, EVs exhibit an enormous molecular complexity turning them into multifaceted signaling vehicles delivering a plethora of different messages, which are integrated in target cells producing varied responses. A growing body of literature on the multiple functions of EVs points to their involvement in processes as diverse as immune regulation, cancer cell metastasis and tissue regeneration [7]. Furthermore, there are indications for possible effects of EVs that accelerate or slow down the process of aging [17, 18]. Similar to other cell types, brain cells (neurons and glia) release EVs, which facilitate cellular communication within the brain and possibly can act even beyond its borders [19, 20]. For example, following brain inflammatory injury astrocyte-derived EVs can enter the peripheral circulation and trigger cytokine production in the liver regulating the immunological response to brain injury [21].
Exosomes: Promoters of neuronal fitness
Studies on EVs released by oligodendrocytes and their role in the interaction with neurons revealed that oligodendrocyte-derived EVs with exosomal characteristics are internalized by neurons, which can make use of the cargo (Figure 1 - left) [22, 23].
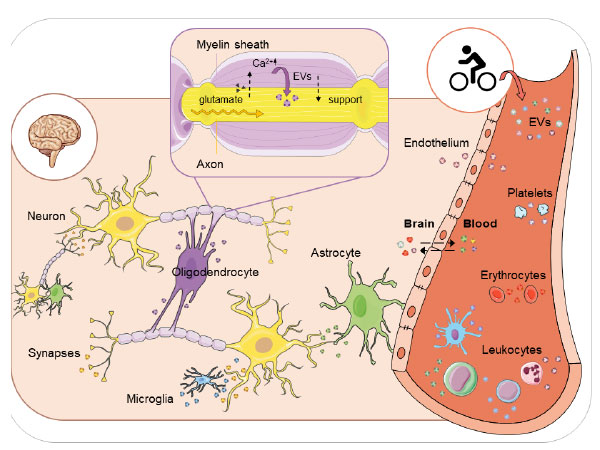
In myelinating oligodendrocytes visualized in cross-sectioned nerves, MVBs most likely encapsulating the future exosomes accumulate in close proximity to the neuronal axon [24]. A series of experiments employing cultured oligodendrocytes and neurons demonstrated that electrical stimulation of neurons is followed by release of the neurotransmitter glutamate and activation of ionotropic glutamate receptors in oligodendrocytes resulting in calcium influx, triggering the secretion of oligodendroglial exosomes from MVBs. Oligodendroglial exosomes are further internalized by the neurons via endocytosis, which can occur at cell bodies as well as axons [24]. Importantly, instead of degradation of the vesicle content after endocytosis in the lysosomal compartment, at least part of the EV-cargo is recovered. The biological activity of the exosomal cargo was proven by manufacturing oligodendroglial EVs carrying a reporter enzyme (Cre-recombinase) that can activate a marker gene in target neurons (e. g. LacZ or EGFP) upon retrieval from internalized EVs. Treating cultured neurons with Cre-carrying EVs or injecting Cre-carrying EVs into the brain indeed resulted in recombined neurons in culture or in the injected brain regions, respectively [24]. Thus, electrically active neurons stimulate myelinating oligodendrocytes to release EVs, which at least partly consist of MVB-derived exosomes, and these EVs deliver functional cargo to neurons.
What may be the function of EV-transfer between oligodendrocytes and neurons and do neurons benefit from the uptake of oligodendroglial EVs? A working hypothesis is that glia to neuron EV-transfer provides molecular support to areas of the axons, which are located far from the neurons' cell body, and helps them to maintain homeostasis. The transmission of electrical signals produced by ion currents requires a lot of energy and furthermore, promotes the formation of harmful oxygen radicals [25]. This leads to acute nutrient deficiency and oxidative stress. In vitro examinations revealed that treatment with isolated oligodendroglial EVs helps neurons exposed to oxidative stress and nutrient deprivation to cope with these straining conditions. Neurons receiving EVs fire action potentials at higher frequencies and exhibit higher metabolic activity and improved fast axonal transport as compared to neurons not receiving EVs or receiving EVs from another cellular source [24, 26]. Moreover, when oligodendroglial EV-release is analyzed in PLP- and CNP-deficient mice, where axonal degeneration occurs secondary to oligodendroglial defects, apparently EV-release is reduced by approximately half and the EV-content is altered. Most intriguingly, aberrant EVs derived from these mouse mutants have lost the functional ability to maintain the neuronal metabolic activity and do not promote axonal transport [26]. Hence, oligodendroglial EVs mediate the maintenance of vital cellular activities in neurons under highly demanding conditions in favour of general neuronal fitness, essential for neuronal long-term maintenance across the lifespan.
Interestingly, the electrical activity in neurons itself stimulates their cellular maintenance facilitated by oligodendroglial EVs. Thus, neurons receive glial support in form of membrane-protected bioactive cargo “upon demand” at local sites of high workload associated with stress exposure. While the exact mechanisms, by which EVs positively influence neuronal functions, and the molecules involved need to be further elucidated, they appear to be part of a complex intercellular communication pathway responsible for long-lasting neuronal resilience and fitness.
Throughout the brain, EVs are involved in the maintenance of homeostasis as well as in local adaptation (neuronal plasticity) and regeneration processes. Indeed, various other inter-cellular communication processes in the brain, which are affected by EVs are similarly regulated by neural activity [20, 27, 28]. However, in contrast to their attested neuroprotective properties, EVs are also believed to contribute to the propagation of key pathogenic proteins in neurodegenerative diseases like Alzheimer’s disease. Overall, a complex picture of intercellular exchange is evolving, involving various molecules packed and transported within different types of EVs not only acting within the brain but also reaching across its barriers.
EVs in physical exercise: Benefits for neural health?
Regular physical exercise is one of the most important recommendations for a long and healthy life. It is well accepted that, under controlled conditions, exercise leads to physiological adaptations, which have a positive effect on processes like endothelial function, immune signaling and neuroprotection. And, on a long-term, these adaptations lead to improved physical and mental health. The latter is characterized by improved neurogenesis and synaptic plasticity [29, 30]. In the aging process, this leads to a reduced decline in age-related impairments: Active individuals are less prone to develop Alzheimer's and Parkinson's disease, and disease progression is reduced in exercising patients [31]. In addition, exercise reduces cognitive decline and improves quality of life in depressed patients [32, 33]. While a positive effect of physical activity on the general state of health is well documented, the exact molecular basis is still not fully understood. However, there is increasing evidence that EV-based tissue crosstalk may underlie the adaptive processes following exercise [34].
Interestingly, a single bout of physical exercise not only triggers a broad change in physiological factors, but also mobilizes EVs into the circulation (Figure 1 - right). These appear at an early stage of exertion before acidosis [35, 36]. Thus, this effect does not seem to be a direct result of the metabolic stress caused by oxygen debt, but rather an active signaling mechanism. Cells associated with the circulatory system (endothelial cells, platelets, leukocytes) as well as muscle cells were identified as the originating cell populations of EVs, which are released in diverse exercise settings [36, 37]. Cargo analysis of the released EVs suggested the transport of myokines and myomiRs indicating muscle cell signalling, maybe in terms of regenerative processes [37–39]. In addition, a multitude of proteins relevant for adaptive processes in response to physical exercise (formation of new blood vessels, immune signalling, glycolysis, among others) were detected in EVs released during exercise [36, 37, 40]. According to various studies, EVs from the indicated cell types participate in the regulation of immune and inflammatory processes, have a protective influence on the cardiovascular system, including endothelial function and blood vessel-formation, and also act on the brain [7]. Further research is ongoing, addressing a possible EV-based organ interplay involved in adaptation processes triggered by exercise, which might promote physical and mental health.
Under physiologic conditions, the central nervous system is protected from insult from the circulation by a barrier, which controls selective exchange of substances between blood and brain. Accumulating data suggest that EVs can overcome the blood-brain-barrier (BBB) and contribute to signaling processes between the periphery and neuronal cells. Recently, it was demonstrated in vivo that EVs originating from the hematopoietic lineage can cross the BBB and transfer bioactive cargo to recipient neuronal cells, which seems to be enhanced under inflammatory conditions and by neuronal activity [41, 42]. Likewise, EV-mediated cell-cell communication at the blood-cerebrospinal fluid-barrier appears to be part of the immune reaction at the interface between neural tissue and the periphery [43]). Observations like the ability of peripherally administered EVs to pass the BBB and induce glial cell activation [44] additionally raised interest in the therapeutic and regenerative potential of peripheral EVs, including mesenchymal stromal cell (MSC)-derived EVs [45–47]. Further research will be needed to define the distinct EV signaling routes between blood and brain as well as the role of inflammation and physical exercise in this context. Intriguingly, acute exercise bouts increase the BBB permeability, which in long-term is suggested to induce protective adaptation mechanisms that lead to enhanced BBB integrity, e. g. via anti-inflammatory and anti-oxidative signaling [48]. It will be interesting to examine the influence of exercise-derived EVs on the BBB characteristics and their potential beneficial impact on neuronal health and the process of aging.
Conclusion
Healthy brain performance requires optimized communication between neurons and glial cells as well as exchange of signals with the periphery. A sophisticated and directed mode of cell-to-cell communication is facilitated by EVs, which transport membrane-protected, bioactive cargo between cells and through body fluids. Transfer of oligodendroglial EVs within the axon-myelin unit plays an important role in the support of axonal maintenance, especially at sites far away from the neuronal cell body. Interestingly, this form of neuronal maintenance and long-term protection is driven by neuronal activity itself. Similarly, physical activity leads to a liberation of EVs into the circulation, possibly in the context of homeostasis as well as tissue regeneration, and is also associated with neuronal health. The extent to which the diverse EV populations from cells within the brain or from the periphery actually mediate neuroprotection remains to be investigated. Thus far, the recommendation is to stay physically and mentally active and exercise “EV brainstorming” to keep our neurons lively and healthy.
Acknowledgements
We thank Prof. Dr. Dr. Perikles Simon for continuous exchange and tireless discussions.