On the Interplay of Extracellular Vesicles and Viral Infections
road definition of extracellular vesicles – lipid membrane enclosed vesicles of a given size range, produced by cells into the surrounding media and unable to replicate independently – does not only apply to exosomes or microvesicles produced by eukaryotic cells, outer membrane or outer-inner membrane vesicles produced by gram-negative bacteria and membrane vesicles produced by gram-positive bacteria (and archaea), but also extends to enveloped virus particles. They share biophysical and biochemical characteristics as well as functional properties, making it a challenge to distinguish between types of vesicles.
Keywords: Virus, viral particles, exosomes
Introduction
Definition and Types
From humble beginnings, extracellular vesicles (EVs) have come a long way. Recently, not only basic science has shown strong interest, since numerous physiological functions have been reported and are still being reported [1–7], but also the first applications in biomedicine are emerging, mostly in diagnostics [8], but also in gene therapy [9] and vaccine development [10]. However, the field remains in its infancy, with concomitant problems [11]. A general definition of EVs includes all lipid bilayer-membrane enclosed vesicles produced by cells unable to replicate independently, thus also encompassing enveloped viral particles (e. g. human immunodeficiency virus, HIV; Influenza; Severe acute respiratory syndrome-Coronavirus 2, SARS-CoV2). The viral envelope constitutes the lipid bilayer lining, which covers the viral protein capsid, which in turn contains the viral genome. Non-enveloped (or “naked”) viruses are not considered EVs but still might interact with EVs [12].
Before concentrating on eukaryotic EVs, we will make a brief detour to prokaryotic organisms. Both gram+ and gram- bacteria produce EVs [6, 13–16] which contribute to functions as diverse as quorum-sensing, horizontal gene transfer, expulsion of unwanted material, or communication in microbial consortia, as well as mediating host-pathogen interactions [13, 14, 16]. Also Archaea produce EVs, first described in Sulfolobus islandicus [17]. A full treatise on prokaryotic vesicles would exceed the scope of this review, however recent overview articles are available [6, 14, 18, 19]. EVs in eukaryotes can be sub-grouped either as ecto- or endosomes [3, 20, 21]. Ectosomal vesicles are generated at the cell membrane and shed directly into the surrounding environment – a process sometimes termed ectocytosis [22]. This group includes microvesicles, apoptotic vesicles and other specialized vesicles such as migrasomes [23] and oncosomes [24]. On the contrary, vesicles from endosomal origin are produced into the lumen of endosomes, generating a multi-vesicular body (MVB). Upon exocytosis the stored EVs are released. Exosomes are the main example in this category. Classification is complicated by the use of trivial names based on cellular origins (e. g. prostasomes, migrasomes) [7]. We will adhere to the definition of EVs given above and refer to specific types were indicated.
Functions
Table 1 and Figure 1 give an overview of the types of EVs in different organisms and list key properties, potential marker proteins and main functions (e. g. cellular communication, waste disposal, horizontal gene transfer and immunity modulation).
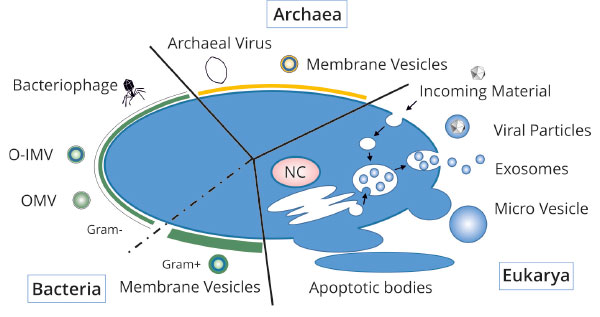
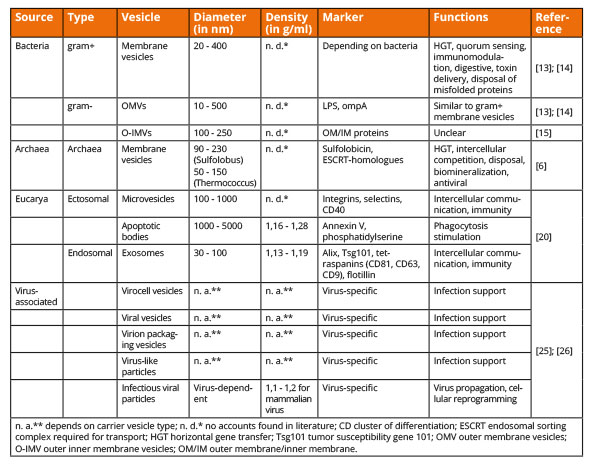
Under physiological conditions, the main function of EVs is bio-molecule delivery and subsequently signal-transduction to target cells. EV transferred proteins and nucleic acid molecules find their interaction partners on or in the recipient cell. A concept reminiscent of tegument structures (a protein rich matrix located between capsid and envelope) in different virus families (e. g. Herpesviridae). Upon virus entry, tegumental factors become immediately active, converting cells to a more pro-viral state. Such re-programming is also observed after EV uptake into target cells. This cell-to-cell communication is then commissioned for different applications e. g. in development [28], immunity [29], CNS-related functions [30] or more specific tasks i. e. in reproduction [31]. Examples for microvesicles include functions in immunity, hemostasis and angiogenesis. Exosomes have been shown to play a role in reproduction and development [32]. Additionally, a role for exosomes in the maintenance of stem cell niches by promoting cell survival and pluripotency [4, 35] has been suggested.
Also under pathological conditions, bio-molecule delivery and subsequent signaling transduction are considered the major drivers of EV functions. In addition to their role in viral infections (see Part 4), EVs have been implicated in neuropathologies such as prion diseases, Alzheimer’s and Parkinson’s disease, multiple sclerosis [5, 30]. This emphasizes the ability of exosomes to cross the blood-brain-barrier, opening interesting routes for therapy of neurological diseases. In cancer biology, a range of mechanisms involving EVs have been identified [4, 37]. Microvesicles have been implicated in pathologies ranging from atherosclerosis to inflammation, via vascular dysfunction, thrombosis, loss of pregnancy, preeclampsia and sepsis [21]. Exosomes seem to play a role in cancer [2, 37], metabolic diseases (e. g. in diabetes and obesity) [38–40], cardiovascular problems [41] and neuropathologies [42, 43].
In order to perform the signaling functions, vesicles’ composition will need to be adapted to the purpose, making issues of biogenesis and sorting of content to the vesicle a key issue. On the contrary, viral particles have a more clearly defined function: replicate and protect the viral genetic information. Therefore, the content may stay relatively constant.
Biogenesis
Eukaryotic cells contain a complex endomembrane system, encompassing the nuclear envelope as well as the endoplasmatic reticulum, the Golgi apparatus and mitochondria. Additionally, different vesicle types connect these compartments and sort content into the organelles or provide specific functions such as specific degradative operations (i. e. autophagosomes) or cellular exit (exocytotic vesicles, multivesicular bodies, exosomes). Apoptotic bodies and membrane vesicles are generated from ectosomal origins: cell membrane is reorganized as a consequence of intracellular mechanisms and vesicles bud away from the main body of the cell. For microvesicles, the increase of intracellular calcium levels seems to initiate the reorganization of membrane, resulting in rearrangement of membrane. Calcium-sensitive enzymes are activated and drive cytoskeleton changes facilitating vesiculation [21]. For apoptotic bodies, Rho-associated kinase I (ROCK I) and myosin ATPase seem to be involved in vesicle formation [21, 44]. Exosomes are generated from elements of the endosomal compartment, multi vesicular bodies. The process involves endosomal sorting complex required for transport (ESCRT)-dependent and independent elements [21]. ESCRT-dependent mechanisms use amongst others, the ESCRT-0 to III complexes and ALIX [45], while ESCRT-independent processes involve tetraspanins and changes in lipid conformations [21]. However, details are unclear and exosomal biogenesis is discussed controversially. Several intrinsic e. g. adiponectin/
T-cadherin signaling [46] and extrinsic factors e. g. viral or other infections) may influence exosome biogenesis. Other vesiculation-related mechanisms seem to feed into this pathway. Autophagy as a means to overcome cellular starvation may lead to the formation of vesicles, which also contribute to viral transmission of Herpes- and Picornaviridae [47]. Vesicles with exosomal characteristics are also produced directly from the cell membrane, a process reminiscent of HIV budding [48]. Viral assembly (as counterpart to the EV biogenesis) happens at different cellular sites, depending on the virus in question. However, often in close proximity of intracellular membranous structures, even for non-enveloped viruses (e. g. for Picornaviridae, see viralzone.expasy.org/1951). Viruses may exploit different options for cellular exit, either by direct budding from the cell membrane (enveloped virus only), exocytosis or lysis of cells. Additionally cell-to-cell transmission via plasma bridges or cell fusion have been reported. Recently EV-mediated transmission was added to the list [49–51].
Content, Cargo and Delivery
In viral assembly, the genetic material of the virus is labeled by packaging signals, which recruit the RNA or DNA molecules for encapsulation in the virus protein core. Viral proteins carry interaction domains, which may be sufficient for self-assembly of the virus capsid (e. g. Retroviridae), or require assistance from scaffolding molecules (e. g. Herpesviridae). For small RNAs a combination of short structured motifs seem to be important for sorting into EVs [52, 53] and similarly for mRNA [54]. For EV protein content a range of different mechanisms has been discussed. Exosomal cargoes reach MVBs from the Golgi or from membrane recycling [55] and ESCRT complexes may moonlight as exosome fillers [56] as well as tetraspanins [57, 58] and certain types of membrane lipids such as ceramide [52]. Post-translational protein modifications (e. g. ubiquitinylation and SUMOylation) [5] may serve as a packaging signal for EVs. Another study suggests that oligomerization and cell membrane attachment may be sufficient for inclusion in EVs [59].
EVs contain a wide range of bio-macromolecules including proteins (membrane-bound and soluble), DNA and different forms of RNAs (mRNA to small regulatory RNAs) as well as metabolites and lipids that make up the base platform and also play a role beyond providing building blocks i. e. in sorting of proteins to EVs. EV content reflects the biogenesis pathway of the vesicle types as well as the status of the cell. Exosomal protein markers include the tetraspanins CD9, CD63 and CD81, ALIX and TSG101 [21]. In addition, heat shock proteins are commonly found in exosomes. Microvesicles markers include Annexin V and tissue factor (TF) [8, 21] (see Table 1). In terms of nucleic acid content, while mRNA [60] and DNA [61] is detected in EVs, most information is available on small regulatory RNA species such as miRNA which exert their function by regulating mRNA levels in recipient cells. A range of species has been detected and different profiles have been identified in samples derived from pathological samples, strengthening the potential of EVs as diagnostic tools [60]. An early study on mRNAs in EVs derived from glioblastoma cells identified 27,000 mRNAs in the samples – close to 5,000 of these were only found in EVs, not the source cells [62]. Extensive information of proteomic, and genomic/transcriptomic data is available (for example see Vesiclepedia microvesicles.org/databases; ExoCarta www.exocarta.org/), however severely complicated by the inherent heterogeneity of vesicles (from mixed sources) and additional technical heterogeneity (from differing preparation). Contaminating vesicle types (lipopolyproteine, chylomicrons, viruses and virus-like particles) are ubiquitous, especially in serum. In this regard, viruses exhibit a more homogenous setup, since a higher stringency or quality control is necessary to maintain infectivity and most heterogeneity is found in the genome. At least, that is the current paradigm. However the borders between EVs and VP may be blurred and several intermediates were identified: from virocell vesicles (EVs produced by infected cells, even if no viral content is present, may however contain virally modified content), via viral vesicles (EVs containing viral nucleic acids), virion-packaging vesicles (EVs containing virions i. e. in Hepatitis A and E) and virus-like-particles (replication-incompetent virions) [25, 26]. Content may also be engineered to be contained as a ”payload” in EVs, e. g. antigens for vaccine development [11, 63] or therapeutic RNAs for gene therapy [10].
Current research suggests, that all available mechanisms for cell entry may be exploited [21]: Membrane fusion, endocytosis, phagocytosis and macropinocytosis may be employed to internalize EVs.
Practical Issues – A short guide to Culturing, Preparation and Characterization
In Table 2 we provide a brief overview of methods in EV preparation.
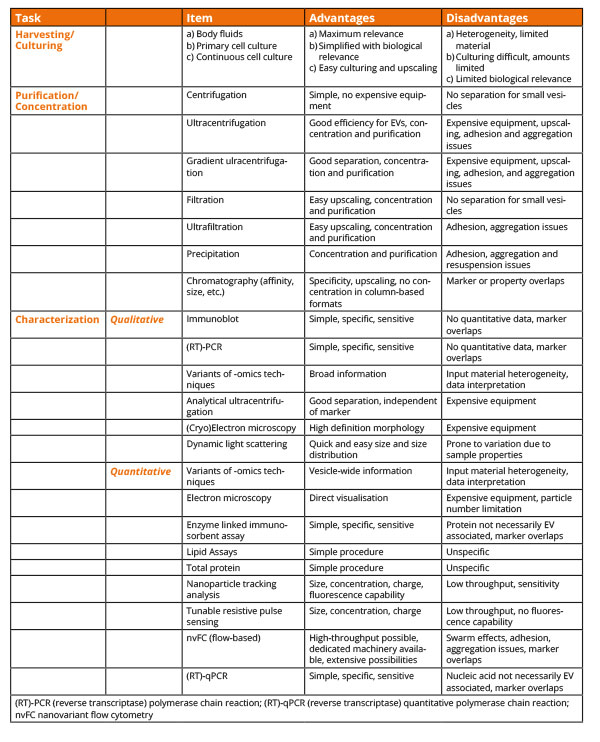
Important general considerations before starting preparation of EVs are the reduction of aggregation and surface adsorption (i. e. by buffer choice, choice of particle density, choice of plastic consumables), and the use of aggregate- and vesicle-free material where possible (pre-purified serum, buffers, etc.).
EVs may be derived from serum or other body fluids, primary culture or continuous cell culture systems. Except for the last method, were scale-up is less of an issue, getting significant amount of raw material is a problem. Chemicals for the stimulation (e. g. monensin) [27] and inhibition (e. g. GW4869) [28] of EV generation are available and starving or treating with toxins/antibiotics may increase vesiculation, probably by engaging autophagy [29]. However, due to the vesicles’ dynamic nature such procedures will most likely change composition. Two goals need to be accomplished: removal of contaminating elements and reduction of the volume to increase the concentration of EVs. Each additional step to improve purity/concentration will reduce the yield to a degree. Therefore, purity and yield will be kept at a level sufficient for the planned research. Preparative strategies follow a common pattern: crude contaminants (cells, cell debris and larger aggregates) are removed first by a low-speed centrifugation step. Then, increasing the centrifugal force will allow fractionation of vesicles, up to the pelleting of EVs by ultracentrifugation (UC). Density gradients will increase discriminatory power. UC provides purification and concentration in one process step, similar to ultrafiltration. Additionally different variants of chromatography techniques are in use including affinity and size exclusion chromatography. Finally, precipitation techniques, such as ammonium sulfate precipitation also play a role [18].
For the qualitative characterization of EVs, commonly used techniques include immunoblotting to detect protein markers of EVs, conventional polymerase chain reaction (PCR) or reverse-transcriptase (RT)-PCR to detect DNA or RNA in the samples, and electron microscopy to show morphology of preparations. For quantitative analysis also a set of methods is available: total protein assays (e. g. Bradford or Lowry-type), total lipid assays, enzyme-linked immunosorbent assays (ELISA), specific DNA (e. g. by quantitative PCR, qPCR) and RNA analysis (e. g. by reverse-transcription qPCR, RT-qPCR), and particle based methods (electron microscopy EM, nanoparticle tracking analysis NTA; tunable resistive pulse sensing TRPS; nano-variant flow cytometry nvFC) [30]. The property the latter share is that analysis is taking place on a single particle level, i. e. we can assign a distinct parameter (size, charge, marker presence) to a single particle, rather than to the ensemble of particles. This allows identifying trait distribution and subsequently heterogeneity in populations and quantifying presence of marker molecules. Methods are based on light-scattering (NTA, nvFC) or the Coulter principle (TRPS). Most promising in this area is the use of flow cytometry adapted for sub-micrometer sized particles; nanovariant Flow Cytometry (nvFC) [31, 32]. Aggregation and adsorption to surfaces also constitute major drawbacks in this area. In addition, swarm effects (groups of small vesicles may be mistaken for a single, larger element, i. e. a cell) need to be controlled for. Cytometers specifically designed for EV analysis are available [33]. For viral preparations, infectivity is another measure of virus titer (e. g. by transduction efficiency or plaque assays). Quantitation of biological nano-vesicles remains a cautionary tale Quantifying by measuring different components of EVs (e. g. protein, lipid, RNA) will differ quite significantly from particle- or function-based data [30].
Interaction of Extracellular Vesicles and Virus Particles
Classical EVs and enveloped viral particles share several characteristics, including size, density and surface markers (see Table 1). In addition, their respective life cycles show parallels: attachment and entry to cells, replication of genomes and expression of proteins, assembly of novel particles and finally exit from the infected cell are the standard steps in viral propagation. Biogenesis of EVs (homologous to assembly) is pre-dated by expression of proteins and replication or transcription of nucleic acids to serve as EV cargos. As for viruses, different exit strategies may be used (exocytosis for exosomes; membrane shedding – a process reminiscent of viral budding for ectosomes). Finding the right target and entering the naïve target cell is mediated by surface receptors, similar to viral infection. Additionally, it is known for a long time, that virus replication (even of non-enveloped virus) interacts heavily with intracellular membranes and thus the cellular vesiculation systems, i. e. in the generation of virions as viral factories (e. g. Picorna- or Flaviviridae, see also viralzone.expasy.org/1951) or by providing the lipid bilayer for viral envelopes. This interplay of viral particles with other types of vesicles or parts of cellular membranes is not only observed in eukarya, but also in bacteria and archaea [6], indicating a deeply rooted function of the EV-VP connection as well as an extraordinary long-standing co-evolution process. Only in the recent years, with EVs becoming somewhat of a research focus has the importance of the viral relationships to cellular membranes been extended to the extracellular compartment. While viruses entering the cells via endocytosis such as members of the family Flaviviridae will become part of the endosomal system, thus most likely to interact with exosome biology, ectosomal interactions have also been reported [34, 35]. An overview of reports on the relationships between extracellular vesicles and viral particles is given in Figure 2 and Table 3.
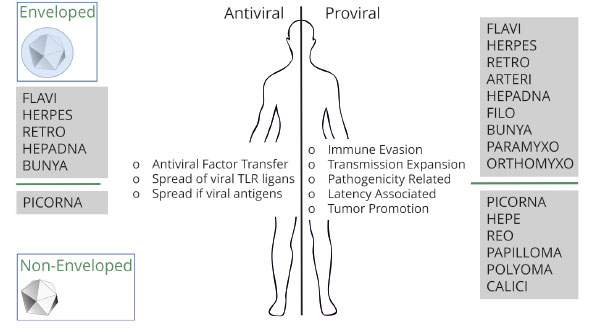
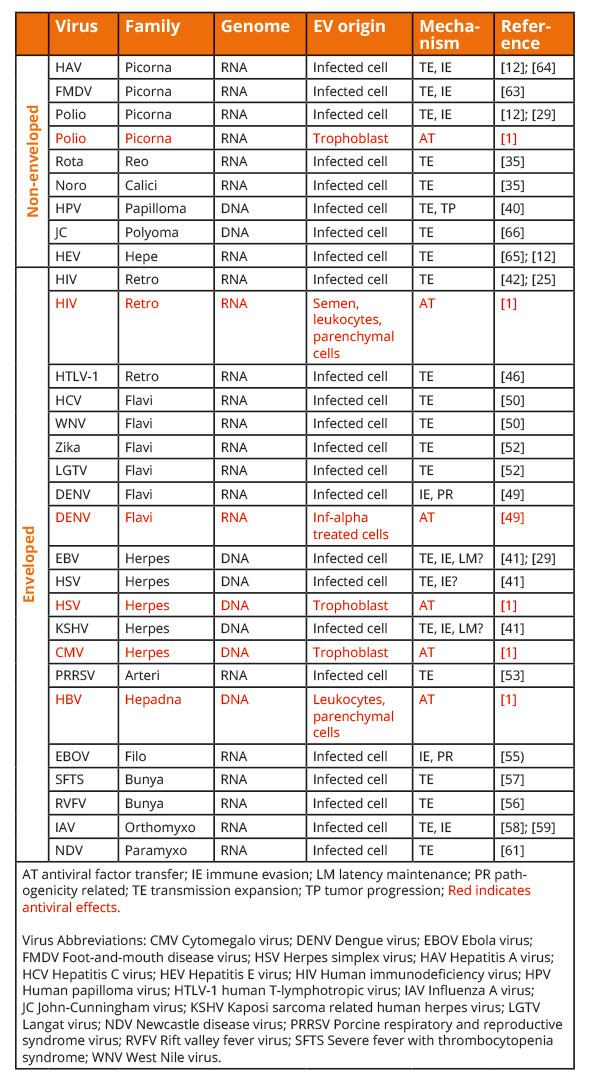
When considering the interactions of viruses, we need to make two distinctions; first, the relationships with enveloped viruses, already containing a lipid bilayer membrane will show different aspects and provide assistance in different areas from EVs than non-enveloped virus particles. Second, the outcome of the interaction may be beneficial (pro-viral) or detrimental (anti-viral) in nature. Pro-viral action includes support in transmission (by different means: from preparing naïve cells for infection, via exploiting additional attachment molecules to full cloaking in EVs), immune evasion, pathogenicity or latency [25, 36–39]. As a side effect, tumor formation may be supported [40]. EV actions may increase the pool of target cells, compromise functions or survival of anti-viral immune cells and increase virus binding to targets. Anti-viral properties have been primarily described for EVs derived from specialized cellular settings (e. g. semen, trophoblast) [1] and exploit strategies such as supplying cells with antiviral components, spreading toll-like receptor (TLR) ligands to augment an antiviral state or transferring antigens to activate immune cells [25]. Distinct functions of the EV-VP relationship may be difficult to isolate: Cloaking of virions in EVs will in most cases make immune recognition more difficult. Similarly, the transfer of antiviral factors between cells will trigger in most cases different arms of immunity.
It has also been discussed, whether a differentiation of virus particles and viral-component modified EVs actually is biologically relevant at least for certain virus types i. e. HIV. In cells infected with HIV exosomes, containing viral factors will be produced as well as non-infectious or non-replicating virus-like particles and fully infectious particles [25]. Essentially, we may have several vesicle population contributing to viral spread by performing different roles. More generally, EVs may be seen as an extension of viral replication mechanisms, providing alternative routes for transmission, regulation of latency as well as pathogenicity, and immune evasion.
Enveloped virus
The presence of a lipid outer shell is common and found in a broad range of viral families, both containing RNA (e. g. Flavi-, Corona- and Retroviridae) and DNA (e. g. Herpes-, Hepadna- and Poxviridae) genomes. The envelope is used as a means of protection, to display viral proteins and facilitate fusion or endocytosis. In addition to viral proteins, a set of cellular proteins may be incorporated. Viral surface glycoproteins may form a more or less dense molecular lattice around the envelope (and subsequently reduce free membrane space or opportunity to incorporate cellular proteins). The spectrum ranges from Retroviridae (containing plenty of cellular protein and relative little viral protein in the envelope) to Flaviviridae (containing a dense, envelope-bound protein layer – a pseudo-capsid - leaving little space for cellular proteins). In such cases even enveloped viruses may benefit from the additional surface molecules (and potential facilitators of cell entry) provided by EVs. Other benefits of recruiting or using EVs is mainly in immune evasion: viral glycoproteins presented on EV surfaces can sequester antibodies and redirect complement. Additionally, involvement in regulation of latency has been described [41].
Retroviridae are a family of viruses using a step of reverse transcription to produce a DNA genome from the RNA genome present in virus particles, which then integrates into the host genome. The model virus and causative agent of acquired immunodeficiency syndrome (AIDS) is human immunodeficiency virus (HIV). A special place seems to be reserved for Retroviruses, which due to the integration and concomitant latency have a very long-lived and intense relationship with their host. The Trojan exosome hypothesis suggested, that HIV particles hijack exosomal biogenesis to produce envelope-like structures [42]. While the overlaps between retrovirus and EV biology are astonishing, classical EV biogenesis seems not to be involved in HIV assembly, since HIV particles bud from the cell membrane (by ectocytosis, rather than exocytosis) [43]. However, ectocytosis is atypical, as factors usually found in exosomal biogenesis and release also have a role in HIV budding [43]. Proviral effects of EVs in HIV infections have been discussed regarding the transfer of accessory proteins (such as Vif, which interferes with antiviral responses or Nef, which promotes the generation of susceptible cells) [25, 42, 44], pathological effects during latency may be caused by EVs in the absence of significant virus production. HIV infection changes the set of miRNAs in cells [37] which appears to be represented in EVs and might have both pro- and antiviral effects. Antiviral effects have been reported for HIV, when EVs derived from human semen and trophoblasts [43]. This may suggest that generation or EV from special niche cells, providing rather specific content from a specific supplement of human cells is generating antiviral responses. However, general mechanisms may be underlying, such as the promotion of interferon responses. In addition, EVs derived from symbiotic vaginal lactobacilli inhibit HIV-1 infection [5] by reducing attachment, indicating the flexibility and universal nature of EV biology. Furthermore, the presence of large quantities of retroviral sequences in the human genome, the so-called human endogenous retroviruses (HERVs) [45] is also suggestive, and EVs might play a role in activation or mobility of HERVs. HTLV-1 is another retrovirus associated with EV activity [46].
Herpesviridae are large DNA viruses (150-200 nm diameter, 120 – 240 kbp genome size) which are characterized by a pronounced propensity for latent stages. Members of the family causing disease in humans include Herpes Simplex- (HSV), Ebstein-Barr- (EBV), Cytomegalo- (CMV), and Kaposi-Sarcoma-related human herpesvirus (KSHV). Herpesviruses are also associated with tumor formation, especially EBV and KHSV [47, 48]. Both EBV and KHSV proteins are found in EVs. EBV protein LMP1 (required for B cell transformation) associates with the tetraspanin (and exosome marker) CD63 and is enriched in lipid rafts, suggesting mechanisms for sorting of LMP1 to EVs. LMP1 inhibits T cell proliferation and NK cell cytotoxicity, both reducing antiviral immunity. Additionally, LMP1 also induces and recruits signaling proteins such as epidermal growth factor receptor (EGFR) to EVs. In this case, EVs may not only be exploited for virus propagation, but also to promote tumor formation. KSHV and EBV, like the majority of herpesviruses, also contain viral miRNAs, which also find their way into EVs, together with sets of cellular miRNAs. In herpesviral infections miRNAs play a significant role and also have been identifed in viral particles and EVs. These vesicular miRNAs have been implicated in multiple functions including maintaining latency, e. g. for EBV [41].
Flaviviridae constitute a group of small, enveloped RNA viruses (50 nm diameter), containing human pathogens such as Hepatitis C virus (HCV), West Nile- (WNV), Dengue- (DENV) [49], Zika (ZIKV). Hepatitis C hides in EVs for immune evasion [37]. The tetraspanin CD81, an exosomal marker protein and a HCV receptor mediate the interaction. In which form HCV is linked to EVs (partial vs. full virion) remains unclear. HCV RNAs were shown to co-localise with exosomal markers CD63 and CD81 [50, 51]. Again, cloaking by EVs potentially extends the host range of the viral particles. This is of special importance, since a significant part of the family flaviviridae uses arthropode vectors (mosquitos or ticks) for transmission. EVs also seem to play a role in overcoming the species barriers between host and vector by providing alternative means of attachment [52], with interesting corollaries for zoonotic infections. For the model Langat virus (a close relative of the human pathogen tick-borne encephalitis, TBEV) full length viral RNA of both orientations as well as viral proteins were transmitted. In that context, exosomes may also help with neuroinvasion, since RNA was shown to be transferred by EVs from the cells that make up the blood-brain barrier to neurons [52].
Other enveloped viruses implicated in interacting with EVs include family members of the Hepadna- (Hepatitis B virus)[1], Arteri- (Porcine Respiratory and Reproductive Syndrome virus, PRRSV) [53], Filo- (Ebola) [54, 55], Bunya- [56, 57], Ortho- [58–60] and Paramyxoviridae [61]. For Bunyaviridae, immune modulatory and transmission expansion characteristics have been described. Exosomes derived from cells infected with Rift Valley Fever Virus induced apoptosis of immune cells at an early stage (before viral production) [56] and for severe fever with thrombocytopenia syndrome (SFTS) virus receptor independent transmission via CD63+ EVs was demonstrated [57]. In the case of the Orthomyxoviridae, Influenza A virus was shown to modify the EV miRNA content with consequences for antiviral and inflammatory responses [58–60]. Finally, Hepatitis B (Hepadnaviridae) may provide one of the earliest accounts of EV exploitation by virus particles. An extraordinarily large amount of so-called sub-viral particles, containing viral antigen, but no genome, is produced, both in tubular and spherical form. This has been previously used as HBV vaccine candidate and potential vaccine platform [62], indicating the potential for EVs in vaccine development.
Non-enveloped virus
Non-enveloped viruses are somehow limited, as to their means of transmission: Exocytosis and lysis of infected cells are the preferred means of cellular exit. Acquiring a non-classical envelope by hijacking EVs can circumvent this situation. Regarding immune evasion a different mechanism may be employed, rather than using EVs as decoy virus, particles may be cloaked in cellular membranes, reducing exposure of viral antigens. Since in a classical situation, the capsid is presented to the exterior (including the immune systems), relevant antigens are mostly derived from capsid elements. When the capsid is cloaked with the EV membrane, access to antigens is limited, thus reducing immune responses.
Picornaviridae are a group of viruses containing a single stranded RNA genome of + polarity. This allows the use of the viral genome as mRNA, leading directly to translation of viral proteins including the RNA polymerase which generates new virus genomes. As a consequence, viral infection may be established in the presence of viral genome only, without any additional viral factors being present. They are non-enveloped and the family contains a significant number of important human and veterinary pathogens including hepatitis A-, poliomyelitis-, rhino- , coxsackie- and foot-and-mouth-disease virus [63]. Especially for rhino- and poliomyelitis virus, transmission in large packages of up to thousands of viral particles within an EV membrane were documented [34, 35]. Clustering of virus in vesicles may increase the chance of productive infection. Fitness of single virus particles varies considerably, dependent on the genetic modifications introduced during genome replication. This is especially the case for quick mutating RNA viruses, which form quasi-species (a set of newly formed viral particles with slightly deviating genome sequences). Thus having more particles to infect can reduce the risk of infection with a defective virus. Picornaviruses also use autophagy-related vesiculation for exocytosis. Coxsackie, Poliomyelitis and rhinoviral particles seem to be exploiting this pathway [29]. HAV is found in two different forms: in stool, the major route of transmission, non-enveloped particles are found whereas host membranes enveloped forms are blood borne and contribute to viremia. Since EV cloaking protects from neutralizing antibodies (but preferentially stimulating dendritic cells), while remaining infectious, this separation into two forms seems reasonable [64].
Other viruses lacking an envelope have been implicated to connect with EV biology, including members of the families Hepeviridae (Hepatitis E, HEV) [12, 65], Papilloma- (Human Papillomavirus, HPV) [40], Polyoma- (JC-Virus) [66], Reoviridae (Rotavirus) and Caliciviridae (Norovirus) [35]. Rota- and Norovirus cause enteric infections and are shed predominately via the fecal-oral route. For both viruses, lipid-membrane enclosed clusters of particles were found in stool (similar to Picornaviridae). These vesicle-coated clusters remained intact during transmission, achieved a high multiplicity of infection (MOI), and thus caused severe disease. The data suggested that the lipid-coated clusters are more virulent than free virus [35, 67]. In Papillomaviruses levels of the anti-apoptotic factor survivin seems to play a role, most likely via regulation of the major HPV pro-tumor proteins E6 and E7 [40]. Consequently, a pro-tumorigenic state may be favored in the recipient cells.
Discussion and Outlook
On first impression, the situation seems rather confusing. A range of viral families – with quite different genetic, molecular biological and medical background - is co-opting EVs for their means, using different mechanisms and mediator molecules with subsequent consequences for different steps of the viral replication cycle. The methodological issues associated with EVs as well as their inherent heterogeneity is further complicating the matter. Even taken this into consideration, we have begun to view viral infection and especially transmission from a new perspective. The current paradigm, of transfer of a single type of virion particle from an infected to a naïve cell may be a simplification. Alternative routes are available, and alternative mobilization of viral content may be used to modulate immune reactions.
Different relevant topics are applied and evolutionary aspects. In terms of biomedical applications, EVs have been implicated in three areas: (i) as biomarkers in liquid biopsies [8], (ii) as particulate platforms in vaccine development [10] and (iii) as vectors in targeted delivery for gene therapy [9]. EVs used in areas (ii) and (iii) are mostly developed in parallel to the use of viral particles: particulate formations of antigens provide an immune-stimulatory advantage and may be augmented either by recombinant incorporation or post-exit modification methods [68] of immune-modulatory factors such as cytokines. Delivery applications use the physiological propensity of EVs to shuttle nucleic acid between cells. Targeting molecules can be integrated to enhance delivery efficiency. An advantage of EV based delivery is increased safety compared to viral vectors, due to i. e. less pronounced immunogenicity or toxicity. In addition, as mentioned previously, internalization occurs via different routes, enhancing the chance of cellular uptake. Liquid biopsies are based on the fact that cellular changes as a result of different pathologies from viral infection to cancer are also represented in EV populations and may be analysed by EVs from serum or other body fluids, rather than having to access the source tissue or organ. In the context of a viral infection, liquid biopsies may help to identify latent infections.
From another perspective, the exchange of EVs and their content between cells seems to be quite promiscuous, allowing transfer between different cell types and organs, species and even between the different kingdoms of life [5, 52]. A universal horizontal gene transfer mechanisms surely constitutes a strong evolutionary driver. An additional evolutionary aspect pertains to the origin of enveloped viruses: similarities in function, biophysical and biochemical properties as well as the shared use of cellular mechanisms may suggest common ancestry. The theory, that an early naked virus initially acquired an envelope by interfering with vesiculation and exit pathways seems a seductive option [69].
Some questions remain open and require researchers’ attention, amongst them sharpening definitions, based on biogenesis and functionalities; deciphering of the EV heterogeneity, also by improved preparation and analytical methods; investigating the fluidity of the spectrum from EV to enveloped virus; and a full assessment of the potential of EVs in biomedicine. Finally, a few words of warning: a sizeable portion of results on EV biology may be compromised by technical issues or conflicting nomenclature, i. e. to assign a given function to a specific subset of EVs will suffer from inconsistencies in preparation and analysis. A critical mind remains the most valuable companion of EV research. Some of the issues have been collected in a recent review article [1] and the associated challenges were identified [69].
In summary, evidence is coalescing, that the production of EVs may constitute a ubiquitous, highly conserved system with significant implications for molecular biology both in physiological and pathological conditions, EVs have been established as a driver of host-pathogen interactions and thus co-evolution, not only for viral infections, but also in driving tumor progression. As such, great importance for pathogenesis and consecutively for the development of anti-viral and more generalized, anti-microbial strategies is connected to EV biology. Horizontal gene transfer by EVs contributes also and may be of special interest regarding the cross-species or cross-kingdom transfer capability [5, 52], which may play a role in zoonotic infections, and more generally constitute a factor in genetic plasticity.