Extracellular vesicles introduction
Extracellular vesicles as gold mine for new diagnostic and therapeutic approaches in medicine
DOI: https://doi.org/10.47184/tev.2019.01.01Extracellular vesicles (EVs) are a heterogeneous group of subcellular particles shed from cells of origin by diverse mechanisms. They carry specific information and are responsible for efficient intercellular communication that is highly important in many physiological processes as well as for the pathogenesis and progression of several diseases. Their unique properties offer the opportunity to use them also for the delivery of therapeutic drugs.
Keywords: extracellular vesicles, biomarker, liquid biopsy, disease detection, prognosis, pre-analytics, analytical requirements
From cells to extracellular vesicles
Cellular diagnostics have been the gold standard for diagnostics of many diseases in laboratory medicine and pathology for many years. Absolute and relative count of blood cells and qualitative features like size, morphology, biochemical and genetic characteristics have been and are still the basis for the diagnosis of a vast variety of diseases ranging from anemia, infections, sepsis, leukemia and lymphoma to coagulation disorders. Similarly, histopathological tissue diagnostics in pathology relies on morphological and molecular biological changes of cells in their organ compounds.
In contrast, subcellular particles have been considered as cellular waste. Likewise non-coding regions in the genome were greatly underestimated until the Encode Project revealed that this abundant genetic material is essential for regulation and fine-tuning of genomic transcription, silencing and activation of genomic regions and repair processes [1]. With the advent of new highly sensitive and high-resolution technologies, research interest in these subcellular particles has increased tremendously and has provided fascinating insights into their structure, biology, cellular release and function. Far beyond „just waste“, their key role for the cell-to-cell communication, the maintenance of the physiological balance within the organism and adaptation to external challenges as well as for the pathogenesis and progression of a plenitude of diseases has been recognized [2].
Heterogeneous group of EVs
Extracellular vesicles (EVs) are considered as particles with a lipid bilayer membrane naturally released from cells [3]. They comprise a wide spectrum of heterogeneous subcellular vesicles and vary greatly in size, density, surface composition, biochemical content, mechanism of formation, release from cells and biological functions that can be used for further sub-classification (Figure 1) [3,4].
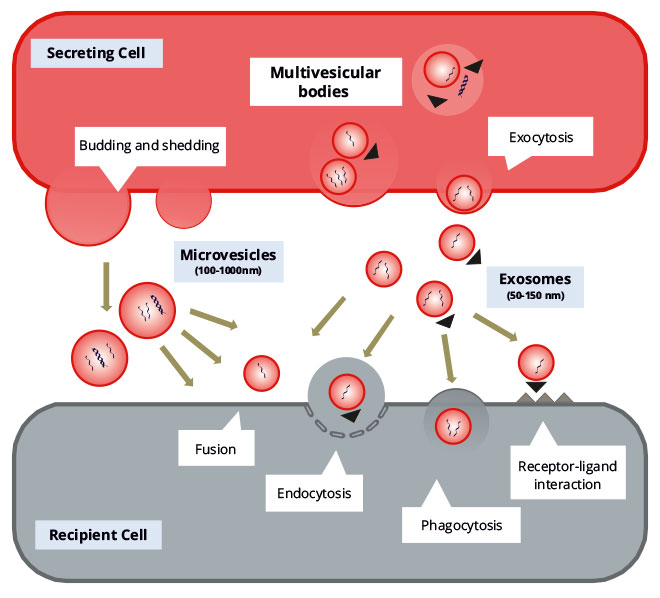
In general, EVs range from 50 to more than 1000 nm in size. Small EVs such as exosomes, which range in size between 50 and 150 nm, originate from a special mechanism of formation in the endosomal system, intracellular maturation within multivesicular bodies and secondary release from cells. In contrast, direct budding from the plasma membrane lead to shedding of larger microvesicles of 100-1000 nm size. In addition, other EVs such as ectosomes, apoptotic bodies and oncosomes are released by specific cell types or dying cells [2–4].
EVs are released in different amounts from healthy, stressed, injured and malignant cells, from platelets, stem cells, cells of the immune system and many more [3,5–7]. They differ in their membrane composition in which transmembrane tetraspanins, proteins, lipid rafts, sugars, nucleic acids, MHC complexes, integrins and other cell type specific markers are incorporated [2,3]. These allow them to exert intercellular contacts with neighboring cells in a paracrine way or even targeted interaction with distant cells after release into the blood stream or other bodily fluids in an endocrine way [3,5,7].
EVs carry different types of cargo (Figure 2).
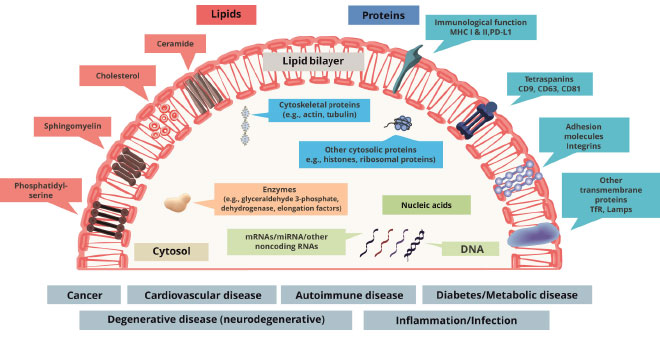
Large microvesicles that are shed from the plasma membrane contain molecules that derive from cytosol, mitochondria, golgi apparatus, endoplasmatic reticulum or even organelles themselves. The mechanisms of specific microvesicle loading are under investigation and it has been shown that certain RNA-binding proteins may traffic into MVs leading to a more specific loading with miRNAs [8]. Endosomal exosomes have a specific cargo mechanism that regulates the specific loading of proteins, lipids, enzymes, DNA, diverse forms of RNA (rRNA, tRNA, mRNA, miRNA and other non-coding RNA), that is dependent (or independent) from endosomal sorting complexes required for transport (ESCRTs) or accessory proteins [2,3,9].
A multitude of EV functions and potential therapeutic applications
After specific binding to the surface of the target cell, endocytosis or fusion with the plasma membrane, this cargo may be released in the cytosol of these cells and modulate there the metabolism, immune or other functions [2,3,5,7]. The exact mechanism how this information is recognized and integrated into the cellular processes has to be further elucidated and understood. Research in recent years has shown that exosomes have excellent performance characteristics as carriers of endogenously or artificially loaded nucleic acids as they are less immunogenic and have a high efficiency when compared to other carriers such as liposomes and nanoparticles [10–12].
In addition, there is an exponential interest in cytoplasmic DNA receptors like cGAS-STING and RNA-receptors like RIG-I, their downstream pathways and links with inflammasome activation, cell death induction and stimulation of exosome release with antitumor activity [13–15]. This opens the avenue for therapeutic use of exosomes as vaccines for infectious diseases or even for targeted delivery of nucleic acid drugs in cancer disease or for tissue regeneration in the heart after stroke [5,11,12,14].
Functional studies have shown that EVs are important mediators of pathogenesis and progression of many diseases. In cancer diseases they are able to transfer malignant information to adjacent and healthy cells leading to transformation, progression, invasion and metastasis of the tumor [7,16]. Thereby, processes like promotion of angiogenesis, downregulation and escape from immune response as well as preparation of a metastatic niche are essential steps for tumor expansion. On the other hand, anti-tumor immune activation may also be mediated by EVs [7,17].
In cardiovascular disease, extracellular vesicles play a role in the crosstalk of various cell types like cardiac muscle cells, fibroblasts and endothelial cells. After hypoxic stress and inflammation they are loaded with different pools of protein or RNA, prevent cardiac damages by attenuating apoptosis and promote cardiac
repair. In other conditions, cardiomyocyte exosomes promote proliferation and
activation of cardiac fibroblasts leading to heart fibrosis and chronic heart failure. After myocardial infarction, exosomes from prestimulated cardiac progenitor cells showed therapeutic potential as they
increased cardiac function and reduced infarct size [5].
Also in other acute disease such as stroke and sepsis as well as in aging and degenerative diseases like Alzheimer’s and Parkinson’s, EVs have shown to play a role in limiting hypoxic damage, fighting infection or promoting chronic disease progression.
Plenty efforts are currently being made to optimize stem cell-derived exosomes
for therapeutic use like in the ischemic brain [18,19]. A more detailed overview on EVs derived from stem cells and their therapeutic use is given in article 5 of this issue.
Challenges in EV detection and characterization
As EVs are heterogeneous and much smaller than cells it is quite challenging to detect, isolate and characterize them sensitively and specifically from bodily fluids, tissues or in functional cell culture studies. Nowadays there are plenty of methodical approaches for isolation, enrichment, quantification and EV- and cell-specific classification. All of them have their strengths and limitations as it is discussed in article 2 of this issue. It is always a trade-off between EV yield and purity that can be obtained and results of studies are strictly dependent on the methods used [3,20,21].
Despite the tremendous growth in publication numbers in the EV field in the recent decade [22], there is still a considerable lack of standardization, data comparability and reproducibility that impairs the better understanding of the biology and function of EVs as well as the translation of results from basic research into patient care [3,20,21]. International and national societies such as the International and the German Society of Extracellular Vesicles (ISEV and GSEV) have been founded to foster the interaction of scientists, the exchange of research experience, and the development of guidelines for the standardized research on EVs in basic and applied science.
One major effort of the international community is the initiative EV Transparent Reporting and Centralizing Knowledgebase (EV-TRACK) that offers a platform for comprehensive, detailed and transparent documentation of EV research and provides an EV-METRIC for adherence to the recommendations [23]. Recently updated ISEV-guidelines on Minimal Information for Studies of EVs (MISEV) [3] is a consensus-based position statement that recommends the appropriate use and precise documentation for methods of EV isolation (separation), enrichment (concentration), quantification and structural and functional characterization. In addition, it clarifies the validation of new EV-associated components and gives assistance for the correct performance of functional studies [3].
As it is difficult to reconstruct the biogenesis of already released EVs they have to be described and classified by size, density, biochemical composition, description of conditions or cells of origin. Therefore, a minimum number of transmembrane/lipid-bound and cytosolic proteins as well as negative protein markers have to be determined to clearly identify EV subclasses and to exclude contamination by exomeres, lipoproteins or other large and abundant proteins which do not exhibit the EV typical lipid bilayer membrane. For functional studies, the topology of a marker outside (secreted) or inside (luminal) the EVs has to be investigated, too. The use of multiple technologies, standards and appropriate controls will strengthen the findings [3].
EVs as biomarkers in various diseases
Beyond pure quantification, specific EV surface markers and the protein, RNA or miRNA pattern of their cargo provide a rich source of information about the cells of origin and EV function [2,5,7,9,21]. If they are released into body fluids such as blood plasma, urine, cerebrospinal fluid, effusions, saliva etc they may serve as sensitive new disease biomarkers as these EV markers are enriched in this specific compartment and reflect the biochemical status of the cells they derive from even if they are far distant.
As in many disease states, thousands of EVs are released from a single cell and they are abundantly found in tissue samples. Thereby, EVs serve an as ideal liquid profiling / liquid biopsy biomarker class outperforming other candidates such as circulating tumor cells (CTCs) or circulating tumor DNA (ctDNA) in terms of sensitivity because they are more rarely detected in patients’ blood. In addition, differentiated analysis of EVs can possibly overcome the difficulties in detecting the heterogeneity of molecular composition and function of cancer cells [7,9,16]. Finally, EVs provide information not only of the diseased cells themselves but also of the response of the host toward these pathologic conditions, e.g. by differentiating of immune suppressive cancer EVs and immune activating EVs of healthy cells [17]. Similarly, complex interactions like in thrombogenesis may be mirrored by EVs deriving from endothelial, immune, cardiac cells and platelets. Therefore, comprehensive analysis of EV surface markers or complete protein and RNA profile by omics technologies will provide important insights [5,6,9,21].
In cancer diseases, EV levels are
considerably increased and can be identified by their surface markers, protein, miRNA and lncRNA patterns. They may be used for diagnosis, estimation of prognosis and disease monitoring as they correlate with therapy response (reviewed in [7,16]). In addition, exosomal PD-L1 was described to contribute to immunosuppression and to be associated with anti-PD-1 response [24].
Likewise in cardiovascular diseases, cardio-specific miRNAs have been found in plasma EVs e.g. after acute myocardial infarction [5]. However, analysis is laborious and time consuming when compared to modern high-sensitive troponin assays limiting its diagnostic value. However, EV-markers may be relevant for estimating prognosis and elucidating the interplay of different cell types.
In sepsis and septic shock, miRNAs are described to be differentially regulated in diverse compartments such as blood cells, serum and exosomes. While plenty of investigated miRNAs were down- and up-regulated, several of them were only changed in serum and/or
exosomes [25]. This indicates that for estimation of diagnosis or prognosis the complex regulative network of miRNA markers in diverse compartments will have to be taken into account.
Remarkably, EVs are also released into the circulatory system in response to physiological changes, such as incremental cycling exercises. Highest EV marker levels were observed at maximum exhaustion with different subtypes deriving from platelets, endothelial cells and leukocytes contributing to the exercise-associated adaptive systemic signaling [26].
While many EV biomarker studies show promising results, many more studies are needed to explore the full potential of the wealth of biomarker candidates in this new compartment for relevant clinical questions.
Analytical requirements for the use of EVs as diagnostic biomarkers
Adherence to guidelines and appropriate documentation is only a very first step to develop robust and reliable biomarkers. As many laboratories are accredited according to ISO 15189 [20] or work according to similar national regulations such as the guideline of the “Bundesärztekammer” (RiliBÄK) in Germany [27], there are strict requirements for the implementation of new markers and methods that are to be used for patient care. By 2022, the new EU In-Vitro Diagnostic Medical Device Regulation (IVDR) will set the stage for medical laboratories on a European level.
All of them require the comprehensive validation of new methods used in medical diagnostics by either manufacturers of a commercial product (followed by a verification by the user) or the medical laboratory itself if the method is an in-house development. This includes high-quality standardization of the methods used, followed by detailed analytical, pre-analytical and clinical validation [20,27].
Analytical evaluation comprises the analytical sensitivity and specificity, accuracy, precision, linearity, measuring range, sample stability and diversity and the uncertainty of measurement [20]. Although this work is less enticing than basic research, it is fundamental for later accurate, reliable and valid use of the methods in patient care and correct interpretation of the results. Particularly the establishment of reference material and implementation of external quality assessment (EQA) schemes – as currently done by certain labs – is essential for quality assurance over methods, users and laboratories [28].
Pre-analytical evaluation considers on the one hand the status of the blood donor like age, sex, body-mass index, pre/postprandial status, exercise level, time of day of collection, current diseases, medication etc. [3,20]. On the other hand, it takes into account all features that affect the sample from blood drawing until later analysis that could affect the measured marker levels, e.g. type of material, container, volume, anticoagulant, time to processing, mode of transport, temperature, exact centrifugation protocols, depletion of platelets and lipoproteins, short- and long-term storage conditions, deep-freezing, thawing, etc. [3,20,29]. Ideally, additional measures to quantify interfering factors such as hemolysis (as in the HIL index), platelet or neutrophil activation that may lead to additional in-vitro release of
exosomes as well as serial venipunctures in individuals to test the biological variation are recommended. In the future,
exosome-preserving tubes (as already available for cfDNA and RNA) and high-quality standards and documentation of pre-analytics (e.g. by SPREC codes) provided by hospital-integrated biobanks may facilitate the pre-analytic procedures. Although cumbersome, all these issues are pivotal for any downstream exosome analysis.
Clinical requirements for the use of EVs as diagnostic biomarkers
Clinical evaluation finally verifies the ability of a method to answer clinical questions, such as (i) the discrimination of diseased from non-diseased persons (diagnosis), (ii) the estimation of the outcome of a diseased person or the risk of disease recurrence (prognosis), (iii) the response to a specific treatment (prediction), (iv) or the serial monitoring of a disease course for early detection of therapy response. For all indications, the methods have to fulfil meaningful user-required specifications [30].
For diagnostic purposes, clinical sensitivity indicates the proportion of correctly identified positive results, while specificity describes the proportion of correctly identified negative results. The overall diagnostic performance is best shown by the complete profile of sensitivity and specificity using receiver operating characteristic (ROC) curves that are able to identify clinically relevant cutoff values to answer clinical questions with an acceptable error rate (e.g. at 95% specificity). However, for clinicians the positive (PPV) and negative predictive values (NPV) may be even more informative as they indicate the probability of disease if the value is positive (PPV) or the absence of disease if the value is negative (NPV). Although these items seem to be sophisticated, they are paramount for the interpretation of any application in patient care. Accordingly, studies with large number of patients and controls in defined conditions have to be performed followed by validation studies in an independent set of samples from another patient cohort [29,30].
Acceptance of EVs as clinical biomarkers
With all analytical, pre-analytical and clinical requirements met, it should not be forgotten that there are some essential points that are extremely relevant for the acceptance in the clinical routine application (Table 1).
1. Analytical performance |
Standardization of methods, calibration, analytical sensitivity and specificity, accuracy, precision, linearity, measuring range, quality controls |
2. Preanalytical performance |
Biological variability within the donor, standardized blood collection and sample handling, materials, tubes, stability against influencing factors (temperature, time etc.) |
3. Clinical performance |
Clinically meaningful EV markers, reference ranges, high clinical sensitivity and specificity, PPV and NPV, interpretable individual changes, dynamics |
4. Routine suitablility |
Clinician: fast, reliable, inexpensive; Laboratory: QC, easy/simple, robust |
5. Future options |
Flexible, portable, scalable |
Table 1:
Requirements to develop EVs as clinical biomarkers
Among others, markers and methods have to:
- answer clinical questions precisely and improve the workflow of the clinicians
- be very robust (low pre-analytic and biological variability), reliable and valid
- be quantifiable and highly quality controlled (internal and external systems)
- be fast and easy to perform (depending on the information and the clinical need)
- be inexpensive and reimbursable
For future wide spread applications they should, in addition,
- be flexible
(for adaptation of new parameters)
- be scalable
(for high throughput automation)
- be portable
(for point-of care diagnostics)
- be miniaturizable
(for mobile and smart devices)
Conclusion
It is obvious that EVs open a wide avenue for future research in cell biology, function and intercellular communication in health and disease. In addition, their properties are ideal for the use of EVs for drug delivery and vaccination. Moreover, EVs provide a fascinating platform for future liquid profiling diagnostics in the blood and bodily fluids that could revolutionize our understanding of both disease and host response. Even if some way has to be made before EVs reach patient care, it is now the time of exploring the EV goldmine right ahead of us.
Acknowledgement
Assistance of Vida Ungerer in proofreading and preparation of the figures is highly appreciated.