EVs from primary neurons
Characterization of extracellular vesicles derived from mouse primary neurons
DOI: https://doi.org/10.47184/tev.2023.01.03It is now clear that the specific composition of extracellular vesicles (EV) is largely determined by the type and developmental or biological state of their donor cells. Careful characterization of EV secretion is therefore important for understanding their functions in specific contexts. Here, we assess neuronal EV secretion, using mouse primary cortical and hippocampal neurons and at two developmental stages. Our proteomics data further shows the presence of widely reported EV proteins such as CD81, Alix and TSG101, and the absence of the commonly used EV marker CD63. Moreover, neuron-derived EVs were significantly enriched in neuronal-enriched proteins, including specific synaptic proteins. Overall, this data provides an initial framework for the isolation of EVs from primary neurons that could be beneficial for specific studies of neuron-derived EVs.
Introduction
Although EVs are thought to be secreted by all cells, cell type-specific characterization of EV secretion is still rather limited. This is important, given the often large differences in gene expression profiles in cells of different origin and developmental stage. Indeed, parallel analysis of EV composition in different cell types and biofluids shows the discrete distribution of EV proteins, including commonly used markers, CD63, CD81 and CD9(Garcia-Martin, Brandao, Thomou, Altindis, & Kahn, 2022; Wiklander et al., 2018). This could be particularly relevant for highly differentiated cell types, such as neurons, that are known to display developmental, regional and functional specificity in gene expression, and that have adapted mechanisms of protein trafficking due to their highly complex morphologies. EVs were previously shown to mediate communication between neurons and non-neuronal cells(Bahrini, Song, Diez, & Hanayama, 2015; Men et al., 2019; Mukherjee et al., 2020), and have been implicated in the spreading of misfolded, aggregating proteins in neurodegenerative disease models(Asai et al., 2015; Lim et al., 2019; Sardar Sinha et al., 2018; Stuendl et al., 2016; Wang et al., 2017). Inter-neuronal transfer of EVs was also shown to be important for the regulation of synapse formation (Bahrini et al., 2015; Lee et al., 2018) and neurotransmission(Antoniou et al., 2023; Vilcaes, Chanaday, & Kavalali, 2021). Here, we evaluate EV secretion from primary neuron-enriched cultures and characterize the protein composition of neuronal-derived EVs using mass spectrometry.
Materials & Methods
List of antibodies
Table 1: Antibodies
Name | Company | Catalogue Number |
---|---|---|
Alix | GeneTex | GTX42812 |
TSG101 | Santa Cruz | sc-7964 |
hnRNPA2B1 | Abcam | ab6102 |
Synaptophysin | Abcam | ab16659 |
Grp75 | Abcam | ab53098 |
Flotilin-2 | Santa Cruz | sc-25507 |
Calnexin | Abcam | ab2301 |
Annexin A1 | Abcam | ab214486 |
Primary neuron culture
Primary cortical and hippocampal neurons were derived from male and female NMRI mice at embryonic day 16 (E16) according to Animal Welfare regulations and as previously described(Antoniou et al., 2023). Neurons were seeded onto cell culture dishes (Falcon) or nitric-acid washed glass coverslips (Carl Roth GmbH) coated with 0,5 mg/ml poly-D-lysine (Sigma) in plating medium (Basal medium Eagle’s containing Eagle’s salts, 0.45 % glucose, 10 % horse serum (Capricorn; HOS-1A), 1 mM sodium pyruvate, 100 U/ml Penicillin and 0,1 mg/ml Streptomycin). Plating medium was replaced with serum-free maintenance medium MEM supplemented with 0.6 % glucose, 0.2 % sodium bicarbonate, 1 mM sodium pyruvate, 2 % B27 supplement (Gibco), 2 mM Glutamax, 100 U/ml Penicillin and 0,1 mg/ml Streptomycin. At 4 days in vitro (DIV), cortical neurons were supplemented with 60 % fresh maintenance medium, which was replaced with every 3 days thereafter. Hippocampal neurons were supplemented with 10 % fresh maintenance medium every 5 days. Neurons were kept in a humidified incubator supplied with 5 % CO2 at 37 ˚C.
EV isolation
Unless otherwise stated, all steps were carried out on ice or at 4 ˚C. Donor neurons were washed three times with pre-warmed maintenance medium before being incubated with pre-warmed fresh maintenance medium for 16 hours at 37 ˚C. For EV characterization by NTA and western blot, 4 million cortical neurons or 1 million hippocampal neurons seeded in 10 cm dishes was used as donors per sample. For proteomics analysis, EV were collected from 12 million cortical neurons per experimental trial. In all cases, the total cell culture supernatant was subjected to differential centrifugation performed as depicted in Figure 1A.
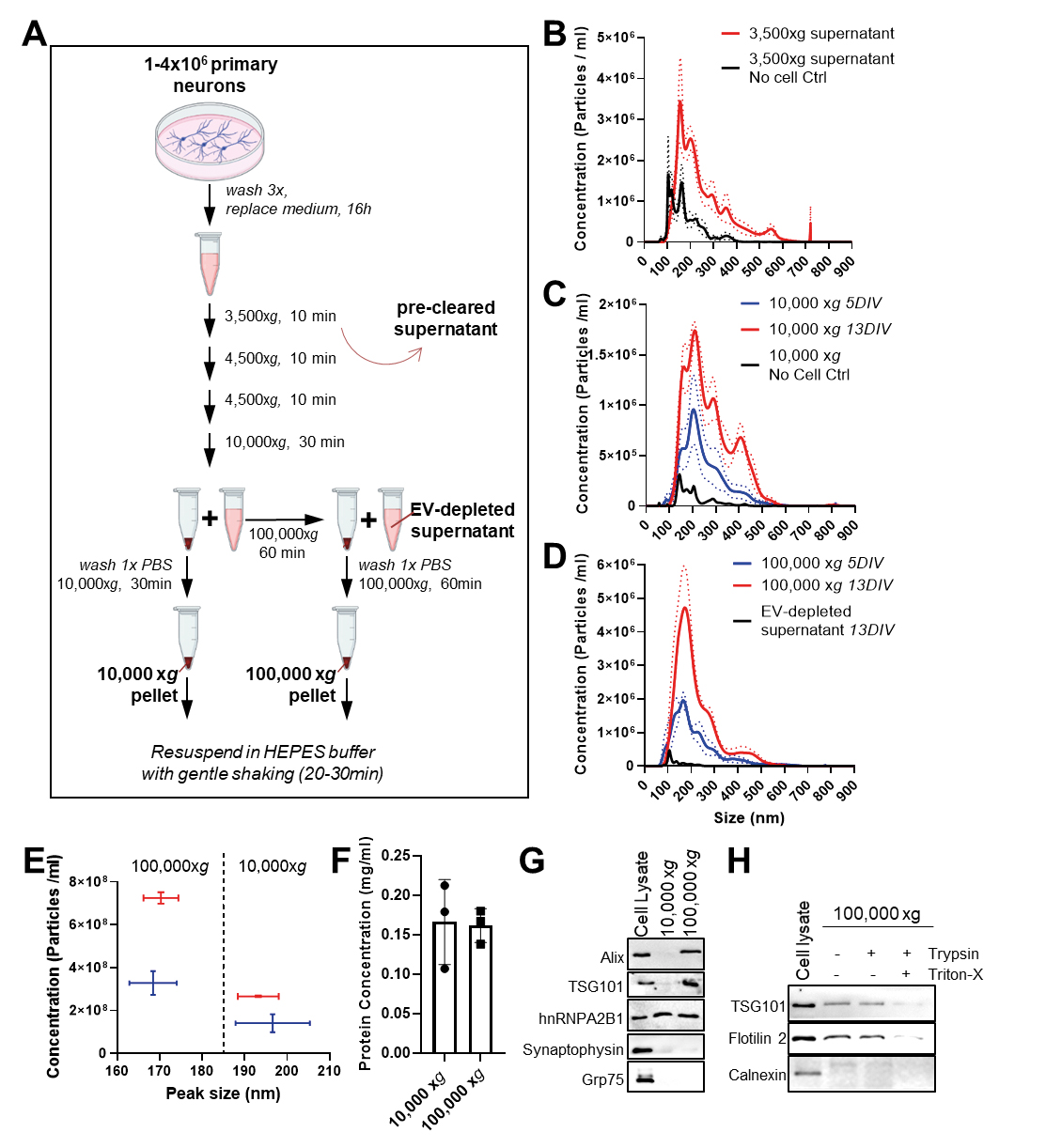
Figure 1: Characterization of EVs derived from cortical neurons and isolated by centrifugation.
A) Diagram depicting EV isolation protocol; for more detail, see methods section. B-D) NTA of 3,500 xg supernatants, 10,000 xg or 100,000 xg pellets derived from 4 million cortical neurons, or no cell controls, as depicted. Dashed lines represent the standard error of the mean; n=5. E) Quantification of particle number and median size of EVs measured as in C and D. Blue; 5 DIV, red; 13 DIV. Error bars represent standard deviation, n=3-5. F) Protein concentration of 10,000 xg or 100,000 xg pellets from 5 DIV cortical neurons, n=3. G) Western blot analysis of cell lysates and corresponding EV pellets. H) Digestion assay of EVs isolated by ultracentrifugation at 100,000 xg.
For isolation of EVs by ultracentrifugation (UC), 10,000 xg supernatants were transferred to thickwall polyallomer tubes (Beckman Coulter) and centrifuged for 1h at 100,000 xg in a fixed-angle rotor (rotor; TLA 100.3, k-factor; 48, Optima MAX-XP tabletop ultracentrifuge, Beckman Coulter). UC supernatants were discarded by decanting. EV pellets were re-suspended in 20 mM HEPES-buffered saline (pH 7.4) containing 0.025 % Tween-20 and protease inhibitors by gentle shaking for 20 min at room temperature. No cell controls were processed in parallel using non-conditioned maintenance media.
For trypsin digestion assay, 100,000 xg pellets were re-suspended in HEPES buffer without protease inhibitors, pooled together and equally distributed across treatment conditions. EVs were treated with a final concentration of 0.008 % Trypsin and 0.09 % Triton X-100 for 5 minutes at 37 ˚C. Untreated samples were treated the same way using HEPES buffer.
EV isolation by immunoprecipitation
Immunoprecipitation was performed using the MACS exosome isolation kit from Miltenyi Biotec (#130-117-039) according to manufacturer instructions. 10,000 xg supernatants from 800,000 hippocampal neurons or no cell control were incubated with 75 µl coupled beads for 90 minutes with rotation at 4 ˚C.
Nanoparticle Tracking Analysis (NTA)
NTA was performed on NanoSight NS500 LM10 instrument and a LM14 viewing unit equipped with a 532 nm laser (NanoSight Ltd). Five 60-second measurements per sample were used for analysis using NTA 2.3 software. For 3,5000 xg
supernatants, approximately 5.7 % of the total medium was collected and diluted 2.5x in PBS. PBS dilution was corrected following acquisition. 10,0000 and 100,000 xg pellets were dissolved in 300 µl PBS and the total sample was processed in NTA.
Protein analysis
Donor cells were washed three times in cold PBS and lysed in CHAPS buffer (1 % CHAPS, 5 mM EDTA and 50 mM Tris-HCl (pH 8)). Post-nuclear supernatants were isolated by centrifugation at 4,500 xg for 10 minutes at 4 ˚C. For quantification of protein concentration, EV pellets were lysed in CHAPS buffer (EVs from 100,000 donor cells/µl CHAPS) by vortexing at full speed for 2 min and cell and EV lysates were subjected to Bicinchonic acid (BCA) assay.
Immunoblotting
Cell lysates or EV pellets were re-suspended in Laemmli sample buffer by vortexing, and heated at 70 ˚C for 10 min before being subjected to SDS-PAGE electrophoresis. Proteins were transferred onto polyvinylidine difluoride (PVDF) membranes, which were blocked in Tris-buffered saline containing 0.1 % Tween-20 and either 4 % semi-skimmed milk powder. Antibodies were diluted in blocking buffer (antibodies used for immunoblotting are shown in Table 1). HRP-conjugated secondary antibodies were purchased from JacksonImmuno Research. Blots were detected using the ChemiDoc MP imaging system (Bio-Rad).
Mass spectrometry
Samples from three biological replicates were processed by mass spectrometry. In each trial, 100,000 xg pellets (approximately 18 µg total protein) were dissolved in 1 % SDS and tip sonicated.
Peptide Preparation
Cleared lysates were subjected to in-solution preparation of peptides on centrifugal filter units modified from (León, Schwämmle, Jensen, & Sprenger, 2013; Manza, Stamer, Ham, Codreanu, & Liebler, 2005; Masuda, Tomita, & Ishihama, 2008; Wiśniewski, Zougman, Nagaraj, & Mann, 2009). Cysteines were reduced with DTT and alkylated with acrylamide followed by buffer exchange (20 mM TEAB, 0.5 % SDC) and digestion with trypsin. SDC was removed from the peptide solution by precipitation and phase transfer with ethyl acetate. Peptides were labeled with isobaric TMTsixplex reagents (Thermo Fisher Scientific, Darmstadt, Germany), desalted on Oasis HLB cartridges (Waters GmbH, Eschborn, Germany) and fractionated with an Offgel fractionator (Agilent Technologies, Waldbronn, Germany).
LC-MS measurements
Peptide separation was performed on a nano HPLC system (Dionex GmbH, Idstein, Germany). 1 µg peptides was injected onto a C18 analytical column (ReproSil-Pur 120 C18-AQ, 1.9 µm) made in-house and separated during a gradient from 5 % o 35 % solvent B (90 % acetonitrile, 0.1 % FA) within 120 min. The nanoHPLC was coupled to an Orbitrap Fusion Lumos mass spectrometer (Thermo Fisher Scientific, Bremen, Germany). Peptide ions were scanned in the Orbitrap detector and fragmented by collision induced dissociation for identification (CID: 0.7 Da isolation, ion trap detection). Fragmented peptide ions were excluded from repeat analysis for 25 s. Top 8 fragment ions were chosen for synchronous precursor selection and fragmented with higher energy CID (HCD: 1.4 Da isolation, 65 % collision energy) for detection of reporter ions in the Orbitrap analyzer (resolution 50,000).
Data analysis
Raw data processing was performed with Proteome Discoverer software 2.4.0.305 (Thermo Fisher Scientific). Peptide identification was done with an in-house Mascot server version 2.6.1 (Matrix Science Ltd, London, UK) searching against murine sequences of SwissProt (release 2019_11, 17027 sequences). Tryptic peptides with up to two missed cleavage sites were searched. Propionamide on cysteines and TMT6-plex on N-termini and lysines were set as static modifications. Oxidation was allowed as dynamic modification of methionine. Mascot results were evaluated by the percolator algorithm version 3.00(Käll, Storey, MacCoss, & Noble, 2008). Spectra with identifications below 1 % q-value were sent to a second round of database search with semitryptic enzyme specificity. Reporter ion intensities were extracted from the MS3 level. Actual FDR values were typically ≤0.8 % (peptide spectrum matches) and ≤1.0 % (peptides). Gene ontology analysis of targets with <60 % variability in abundance was performed in FunRich3.1.3 software(Pathan et al., 2015, 2017).
Results and Discussion
Analysis of secreted fractions from primary cortical neurons
We first investigated neuronal EV secretion from cortical neurons at two developmental stages using nanoparticle tracking analysis (NTA). Pre-cleared cell culture supernatants from developing (5 days in vitro; DIV) cortical neurons revealed that the majority of particles have a peak size of 150 and 200 nm (Fig. 1A-B). Small particles were also observed in unconditioned cell culture media (‘no cell ctrl’), albeit at lower concentrations than conditioned media. We then examined the size distribution of 10,000 xg and 100,000 xg pellets in EVs derived from primary neurons at 5 and 13 DIV, when mature synapses first appear. EV isolation using centrifugation at 10,000 xg or 100,000 xg yielded particles with peak size of approximately 195 and 170 nm, respectively (Fig. 1C-E). Even though protein concentration was similar in both fractions (Fig. 1F), a higher concentration of small EVs isolated by 100,000 xg centrifugation was observed (Fig. 1E). Importantly, the number of particles in no-cell controls was negligible for both fractions (Fig. C-D). Interestingly, EVs collected at 13 DIV had a higher concentration than 5 DIV (Fig. 1E), likely reflecting the membrane expansion in neuronal projections during development. Confirming the presence of EVs in these samples, we could detect TSG101, Alix and hnRNPA2B1 in EV pellets, and the absence of the Golgi resident protein Grp75 (Fig. 1G). In addition, we did not observe the highly expressed synaptic vesicle marker, Synaptophysin in 10,000 or 100,000 xg pellets, thus excluding potential contamination of EV preparations with synaptosomes released from dying cells. Finally, we could confirm the purification of intact EVs using this protocol, as trypsin digestion of intra-luminal EV proteins TSG101 or flotilin-2 was only observed upon permeabilization with Triton X-100 (Fig. 1H).
EV secretion from hippocampal neurons
We next investigated EV secretion from primary hippocampal neuron-enriched cultures. NTA of pre-cleared supernatants revealed a similar size distribution (Fig. 2A) as for cortical neurons (Fig. 1B).
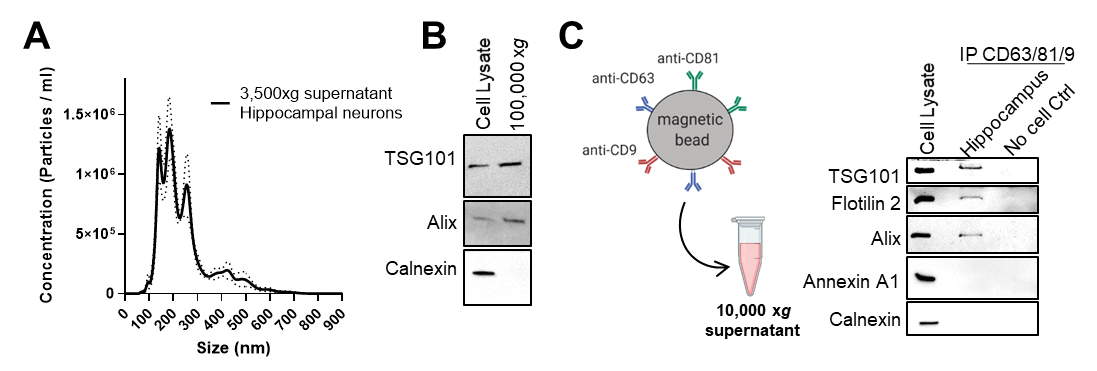
Figure 2: Analysis of EVs derived from primary hippocampal neurons.
A) NTA of 3,500 xg supernatants derived from 1 million hippocampal neurons at 6 DIV. Dashed lines represent the standard error of the mean; n=5. B) Western blot analysis of hippocampal cell lysates and EV pellets as depicted. C) Immunoprecipitation of EVs from 10,000 xg supernatants using magnetic beads conjugated with anti- CD81, CD63 and/or CD9 antibodies.
Particle concentration was approximately four times less than cortical neuron samples, which is consistent to the number of donor cells in each preparation (1x106 vs. 4x106). Moreover, we could detect TSG101 and Alix in 100,000 xg pellets isolated by UC (Fig. 2B), and by immunoprecipitation using magnetic beads coupled to CD81, CD63 and CD9 (Fig. 2C).
Proteomic characterization of neuron-derived EVs
To assess the protein composition of neuron-derived EVs, 100,000 xg pellets derived from 6-7 DIV primary cortical neurons were subjected to liquid chromatography-mass spectrometry (LC-MS) (Supplementary File 1 - use QR-code). Here, we detected a significant representation of the most commonly reported proteins in Vesiclepedia (Pathan et al., 2017) (Fig. 3A, hypergeometric testing, 3.4-fold, *p=2.8e-16). Consistently, there was a significant enrichment of exosome proteins in gene ontology analysis, including CD81, Alix, TSG101 and Syntenin (Fig. 3A, Table 2).
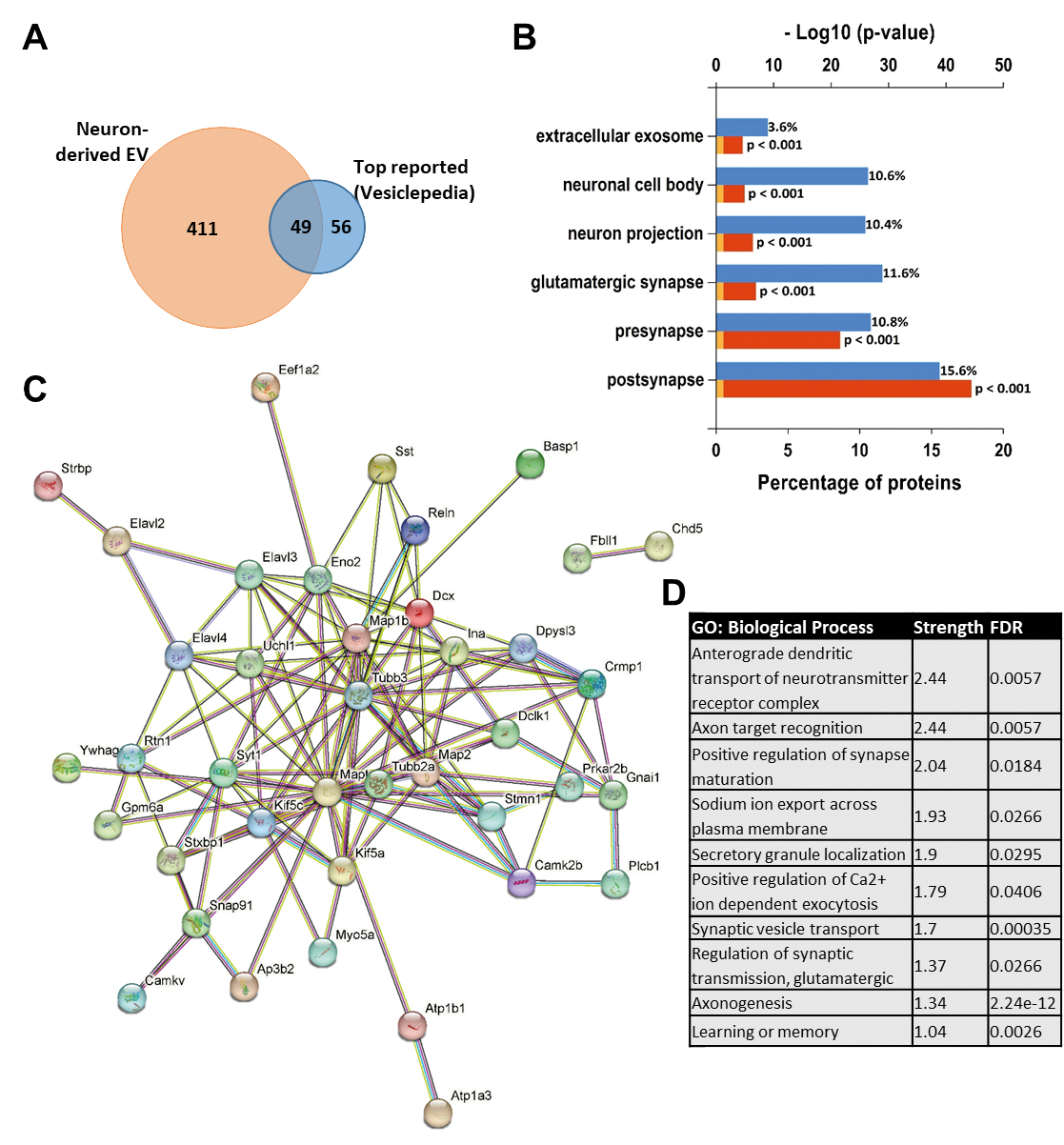
Figure 3: Proteomic analysis of cortical neuron-derived EVs
A) Venn diagram depicting overlap between the top 100 reported EV proteins and neuronal EV proteins identified by LC-MS of 100,000 xg pellets derived from primary cortical neurons in three independent experiments. B) Gene ontology analysis of LC-MS data as in A. Shown is the percentage of annotated proteins compared to the total number of identified proteins in the sample (blue bars), and statistical analysis for enrichment following Bonferroni correction (red bars). C) String network of neuron-enriched EV proteins. D) Gene ontology terms of neuronal EV proteins shown in C.
Table 2: Enrichment scores and mapped proteins for GO terms from neuronal EV pellets.
Cellular component | Fold enrichment | Bonferroni p-value | Uniport Accession |
---|---|---|---|
Extracellular exosome | 5.258381 | 2.12E-05 | P35762; O35490; P62960; P08226; Q9CZD3; P63038; O88569; Q9WU78; Q9CX00; Q61316; P63017; Q61187; P05064; O08992; P63260; O54990; P61226; P07724; |
Neuron cell body | 2.371965 | 1.1E-05 | P62137; P60041; P56959; Q6PIC6; P70296; Q9ERD7; P17183; Q61879; P53994; Q91ZX7; Q04447; P62960; P08226; O08553; O88569; P97427; P62821; O88809; P20357; Q61701; Q7TMK9; Q9JHU4; Q99P72; P63017; Q9R0P9; P07901; Q99104; P13595; P35802; Q78ZA7; Q61548; P31324; Q9D6F9; Q60841; P0CG49; P10637; P33175; P68040; P50396; Q8K0T0; P11499; O08788; P30416; Q9QYR6; P14873; Q6PIE5; Q76MZ3; P27600; P28738; P14206; Q9EQH3; P18760; P62631; |
Neuron projection | 2.640407 | 3.95E-07 | P62137; Q8BG05; Q9R0Y5; P70296; P0DP26; P17182; P17183; Q61879; P50247; Q9CYN9; P15116; P18872; O08553; P84078; O88569; Q8BFZ2; O88809; P20357; P28652; Q99P72; P63017; P07901; Q99104; P13595; P35802; Q61548; Q60841; P54227; P46096; P0CG49; Q923T9; P10637; P62835; Q61768; P33175; P28656; P68040; P50396; P20152; O08788; P30416; Q9QYR6; Q6PIE5; Q9ES97; Q06890; Q76MZ3; P27600; P28738; P63158; P62962; Q9EQH3; P18760; |
Glutamatergic synapse | 2.562415 | 1.05E-07 | P26645; Q9Z2D6; P62137; P63087; Q8BG05; Q60668; P46660; P16858; Q61879; P08226; P15116; Q8BYI9; P63101; P84078; P61750; O08599; B0V2N1; O88569; O08585; P12367; P20357; Q61701; P28652; P84091; P09055; Q9D0E1; Q9DBG3; P39688; P25911; Q04736; Q99P72; P63017; Q99104; P13595; F8VPU2; P35802; P31324; Q64487; P46096; P61979; O54774; P62259; Q9Z1B3; Q99JI6; P62835; Q01853; P60710; P83510; Q9ES97; P58252; Q76MZ3; Q9CVB6; Q8BFZ3; P14206; Q3UHL1; P62962; Q9EQH3; P18760; |
Postsynapse | 8.329752 | 3.43E-45 | Q9Z2D6; Q6ZWV3; P63325; P62137; P63087; P47962; P14148; Q8BG05; Q9D8E6; P46660; Q6PIC6; P62281; P62889; P47963; P53026; Q6ZWN5; Q9CPR4; P28660; Q9CZX8; P19253; P47911; P62849; O08599; P63276; P83882; P35979; P62918; P20357; Q61701; Q9Z2U0; P14115; P84091; P61358; P17426; Q9CZM2; P41105; Q8BP67; Q9DBG3; Q9D1R9; Q99P72; P63017; P60867; Q99104; Q6ZWV7; Q9CR57; P62908; P62855; P17427; P31324; P99027; P14869; P62983; P62984; P61979; O55142; O09167; O54774; P25444; P12970; P62830; P97351; P61255; P62751; P62900; P58252; P62267; P62196; P62082; Q9CVB6; P51410; P62911; P63323; P14206; P14131; Q3UHL1; P62962; Q9EQH3; P62270; |
Presynapse | 5.815138 | 2.3E-22 | Q6ZWV3; P63325; P62137; P63087; P47962; P14148; Q9D8E6; P62281; P47963; P53026; Q9CPR4; P62754; P59279; P19253; P47911; P62849; O08553; O08599; P83882; P62821; P35979; P62918; P14115; P61358; P61982; Q9CZM2; P41105; Q8BP67; Q9D1R9; P63017; P35802; Q6ZWV7; Q9CR57; P62855; P99027; O08992; P62983; P62984; O55142; Q9JME5; O54774; P62835; P12970; P62830; P83510; Q9ES97; P61255; P62751; P51410; P62911; P63323; P14131; P62962; Q9EQH3; |
In addition, we observed the significant enrichment of proteins found at neuronal compartments, particularly in excitatory glutamatergic synapses (Fig. 3A, Table 2). To examine whether neuron-enriched proteins are present in EV preparations, we compared our proteomics data with previously published datasets of cell type-specific proteins in the mouse brain(McKenzie et al., 2018). We observed the significant over-representation of neuron-enriched proteins in our EVs (1.73-fold, *p=6e-4), which are highly enriched for biological functions relating to axonal and dendritic transport, synapse maturation and calcium dependent exocytosis (Fig. 3C-D). Overall, this data suggest that neuronal EVs are involved in synaptic communication between neurons, consistent to previous reports (Antoniou et al., 2023; Ashley et al., 2018; Fauré et al., 2006; Lee et al., 2018; Pastuzyn et al., 2018; Vilcaes et al., 2021), and provide insight into potential candidates that may mediate EV uptake near or at synapses.
Conclusion
The careful characterization of EVs from different cell types is an important step for elucidation of their functions, particularly in multi-cellular tissues and organisms. We anticipate that our pilot data on neuronal EV secretion and protein composition provides an initial framework for further studies on neuron-derived EVs and hope that it helps researchers to navigate this increasingly complex field. Further refinement of neuronal EV isolation, characterization and inter-cellular trafficking will undoubtedly benefit future investigations of ‘brain-derived’ EVs and thus ultimately enhance our understanding of their roles in the nervous system.
Acknowledgements
We thank Julia Lindlar for technical support. Protein identification was performed at the Core Facility Analytical Proteomics, Institute of Biochemistry and Molecular Biology, Medical Faculty, University of Bonn, funded by the Deutsche Forschungsgemeinschaft (DFG, German Research Foundation, project number 386936527). AA receives funding from the DFG (project number 502254462).